Sponsored by HORIBAAug 17 2005
Nanophotonics is one of the most exciting new fields to come out of nanotechnology. The quantum confinement effects implicit in these very small (~ 10 nm) particles can lead to unique optical properties. Rare-earth (RE) doped materials are particularly of interest due to their fluorescence emissions in the visible and infrared regions of the spectrum.
Studying the Fluorescence Properties of RE-Doped Nanopowders
There is an interest in examining the effects of particle size on the fluorescence properties of RE-doped nanopowders as the optical characteristics of RE ions are strongly influenced by their local bonding. Since most photonic devices require these powders be incorporated into a host matrix (i.e. polymer, glass, solvent), there is a need to investigate the emission properties in different host materials. A fully-integrated HORIBA spectroscopy system (sample chamber, Triax 550 monochromator, detectors) was employed to study the effects of different solvents on RE-doped nanopowders.
Synthesizing Rare Earth-Doped Nanopowders with Dopant Concentrations - a Description of the Experimental Process
Optically-active, RE-doped nanopowders containing the rare-earth ions (Er3+,Yb3+) were synthesized with several dopant concentrations. The powders were first analyzed out-of-solution in order to obtain the as prepared fluorescence characteristics. This was done by placing a small amount of powder between two glass slides. Measurements were made in reflectance mode using the SampleMax Solid State Sample Holder (600 gr/mm grating blazed at 1.5 micron) with the sample placed approximately 45° off the entrance slit focal axis. Solid state laser diodes or a Ti:Sapphire ring laser were used to pump the absorption bands of the RE-doped nanopowders.
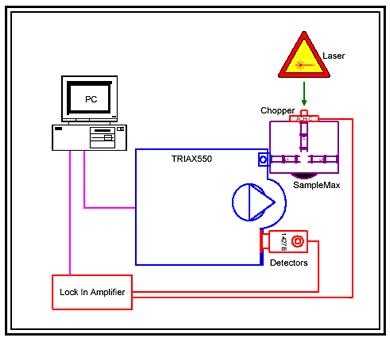
Figure 1. Diagram showing an illustration of the experimental process and the instruments used.
How the Level of Fluorescence was Measured
Fluorescence was measured using HORIBA TEcooled InGaAs and PbS detectors, with the output sent directly to a Stanford Research SR850 lock-in amplifier (using the integrated optical chopper for beam modulation). Subsequently, dilute solutions containing various solvents (methanol, ethanol, and cyclohexane) were prepared for in-solution measurements. The samples were placed in cuvettes then placed into the SampleMax rotating turret for fluorescence measurement.
The Results that Emerged from this Experiment
The fluorescence emissions of the nanopowder/alcohol solutions, even in the most dilute samples, were accurately measured with the constructed system. The high resolution of the system allowed for examination of the effects of the host matrix on the emission characteristics of the RE-doped nanopowders. Small shifts in fluorescence peaks are normally very difficult to see with noisier (lower intensity) signals like the dilute powder/methanol solution. However, due to sensitivity of the HORIBA system, small shifts were easily observed.
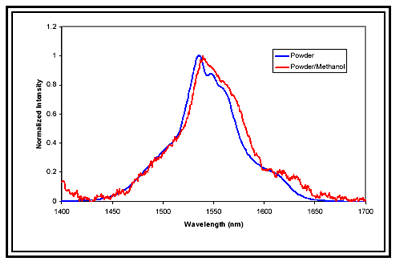
Figure 2. Chart showing Spectra collected with the Jobin Yvon TE Cooled InGaAs Detector.
Conclusion
The HORIBA Triax 550, coupled with the solid state laser diodes, proved valuable to the successful observation of the effects of solvents on RE-doped nanopowders. This high resolution fluorescence system experienced minimal noise, allowing for effective data collection and analysis. The ability to measure liquids, powders, bulk glasses, and thin films in a matter of minutes, was found to drastically increase productivity. The ability to quickly interchange detectors eliminated the need for lengthy alignment procedures when monitoring materials which have emissions across a broad range of wavelengths.
Instruments and Components Used in this Experiment
- Triax 550 Monochromator Triax 550,
- Solid State Detector Interface 1427B,
- Detector, InGaAs, TE Cooled (800 nm–1650 nm) DSS-IGA020T,
- Detector, PbS, TE Cooled (1000 nm–3000 nm) DSS-PBS020T,
- Detector, Silicon, Ambient (200 nm–1100 nm) DSS-S025A,
- SampleMax, Visible ASC-VIS,
- Sample Compartment Turret ASC-STUR,
- SampleMax Optical Rail ASC-ORAIL,
- SampleMax Solid Sample Holder ASC-SSOL,
- Chopper ACH-C,
- Lock-in Amplifier SR850,
- Cable for Silicon Detector +/- 15V CCA-LKDS,

This information has been sourced, reviewed and adapted from materials provided by HORIBA.
For more information on this source, please visit HORIBA.