The tunable microwave-frequency alternating current scanning tunneling microscope (ACSTM) has enabled the recording of local chemical data and local spectra on insulator surfaces, in a similar way to what is now possible using traditional STM for semiconductors and metals.
Spectroscopy in the microwave frequency range helps to realize measurements on conducting substrates that was not possible until now, such as a single adsorbed molecule’s rotational spectroscopy.
The ASTMs Single-Molecule Measurement Methods
The ASTMs single-molecule measurement methods were formulated in the early 1990s by Professor Paul Weiss, the nano-pioneering Director of the Weiss Group, a nanotechnology research unit of UCLA's California NanoSystems Institute.
Using these techniques it has been possible to gain an insight into unprecedented details of chemical behavior, such as the vibration of a single molecule on a surface and the vibration of a single bond in a molecule. These measurements are crucial to understanding entities from single atoms to highly complicated protein assemblies.
The researchers are experienced in determining the exact structures, interactions, environments and functions of molecules at the nanoscale and now trying to understand how to lead molecules into specific positions to develop nanostructures, link functional molecules and the outside world and behave as test structures to determine single or coupled molecules.
David McMillan of the Weiss Group states that the ACSTM allows interactions in and between molecules to be designed, directed, measured, understood and exploited. The group studies how these communications impact dynamics, chemistry, electronic function, structure and other characteristics. These interactions are utilized for creating molecular assemblies, patterns and nanostructures and for controlling and stabilizing function.
By understanding functions, interactions and dynamics at the smallest possible scales, the group is trying to enhance synthetic systems at all scales. The Weiss Group is also utilizing these strategies for controlling and understanding functions, interactions and structures of biological systems.
Understanding ACSTM
The scanning tunneling microscope is founded on the quantum tunnelling principle. Bringing a conducting tip very close to the surface that needs to be examined, a voltage difference applied between them can enable electrons to tunnel through the vacuum between them. The tunnelling current obtained is a function of the applied voltage, tip position and the local density of states of the sample.
Data is obtained by monitoring the current as the position of the tip scans the surface and is shown in image form. Using a STM is quite a challenge as there is need for sharp tips, stable and clean surfaces, advanced electronics and very good vibration control. Good resolution in the case of an STM is 0.1 nm lateral resolution and 0.01 nm depth resolution.
It is possible to regularly manipulate and image individual atoms in materials using this resolution. The STM can be deployed in ultra-vacuum, water, air, several other liquid and gas ambient and at temperatures from near zero Kelvin to a few hundred degrees Celsius.
Very less spectroscopic and chemical data is obtained with atomic force microscopes though they can record topographic information on insulating surfaces. Electrons move to and fro between the tunnelling tip and the sample surface of the microscope. Further to enabling working with non-conductors, the frequency and energy dependences of this transfer process offers chemical data of individual molecular species.
The ACSTM studies insulator surface chemistry using microwaves that are reflected from the surface, microwave throughput attenuation and harmonics of the microwaves generated by the tunnel junction. Photons emitted from the tunnelling junction are determined excited by the tunnelling electrons. This offers considerable gain in spatial resolution as the photons come only from molecules or atoms via which the electrons tunnel through.
Based on testing performed, it is proved that harmonic amplitudes in nonlinear spectroscopy can be interpreted in terms of molecular motions, charging and electronic structure using the ACSTM. These non-linearities have been used by the group to study the electronic energies of insulator surface states.
The ACSTM can study the same surface site for a number of days without contamination or drift, offering information on tunneling conditions over a broad range on the local chemical environment, and on the adsorption site.
Vibration Isolation
In order to attain these nano-level chemical and spectroscopic data sets, the ACSTM must be positioned in a highly stable operating environment, which is free of low-frequency vibrations.
McMillan states that the researchers were mostly using optical tables on pneumatic isolation. A key issue was space constraint. There was a need for smaller pneumatic optical tables however the smaller the table became, the vibration isolation performance reduced.
This was not the only challenge. The lab location was occasionally changed. For instance the sixth floor of a steel-structured building had considerable movement creating low-frequency vibrations. The tables were highly disturbed and thermal stability and vibration isolation became very tough. It was not possible to run the microscopes using the air tables. Hence they started searching for a better vibration isolation solution.
McMillan stated that they tested a negative-stiffness and an active vibration isolation system and the former provided a better performance at the frequencies of concern – low frequencies between 10 and 24 hertz.
Also termed as electronic force cancellation, active vibration isolation makes use of electronics for sensing the motion and then adds electronic forces to cancel or prevent it. Active systems have a limited dynamic range, causing isolator to go to positive feedback mode and cause noise.
The vibration transmissibility of active isolation systems is not as good as with negative-stiffness isolators. Active systems can be influenced negatively by issues with the electrical supply. Heat may also be generated causing thermal drift problems in certain applications.
In Negative-stiffness vibration isolators ertical motion isolation is offered by a stiff spring supporting a weight load along with a negative stiffness mechanism. Without impacting the static load- supporting capacity of the spring the net vertical stiffness is made very low.
Beam columns connected in series with the vertical-motion isolator offer horizontal-motion isolation. The beam-column acts as a spring when integrated with a negative-stiffness mechanism. This helps obtain a compact passive isolator, which can isolate very low vertical and horizontal natural frequencies and very high internal structural frequencies. The frequencies offered by the oscillator are 0.5 Hz* isolation performance vertical, and 0.5 Hz horizontal, using a totally passive mechanical system without any air or electricity.
*Negative-stiffness isolators resonate at 0.5 Hz. There is almost no energy at this frequency. Vibrations having frequencies more than 0.7 Hz (where negative-stiffness isolators begin isolating) are rapidly attenuated with increase in frequency.
There is no need for compressed air or electricity in negative-stiffness isolators. They don't have pumps, motors or chambers and no maintenance as there is nothing to wear out.
Conclusions
The negative stiffness isolator performed better than the optical tables. Transmissibility is also considerably improved using Negative- Stiffness isolators performing about 10 to 100 times better than high-performance air tables depending on the vibration isolation frequencies.
The negative-stiffness isolators, when adjusted to 0.5 Hz, achieve 93 percent isolation efficiency at 2 Hz; 99 percent at 5 Hz; and 99.7 percent at 10 Hz.
About Minus K Technology
Minus K® Technology, Inc. was founded in 1993 to develop, manufacture and market our state-of-the-art vibration isolation products based on our patented negative-stiffness-mechanism technology. Minus K® is based in the Los Angeles area.
Dr. David L. Platus is President and Founder and is the principal inventor of the technology. He earned a B.S. and a Ph.D. in Engineering from UCLA, and a diploma from the Oak Ridge School of (Nuclear) Reactor Technology. Prior to founding Minus K® Technology he worked in the nuclear, aerospace and defense industries conducting and directing analysis and design projects in structural-mechanical systems. He became an independent consultant in 1988. Dr. Platus holds over 20 patents related to shock and vibration isolation.
The Minus K® products, previously sold under the trade name Nano-K®, represent an important enabling technology. By reducing building and floor vibrations to unprecedented levels these systems enable vibration sensitive instruments and equipment to perform at unprecedented levels. They are used in a broad spectrum of applications including nanotechnology, biological sciences, semiconductors, materials research, zero-g simulation of spacecraft, and high-end audio. Minus K® is an OEM supplier to leading manufactures of scanning probe microscopes, micro-hardness testers and other vibration-sensitive instruments and equipment. Minus K® customers include private companies and more than 300 leading universities and government laboratories in 48 countries.
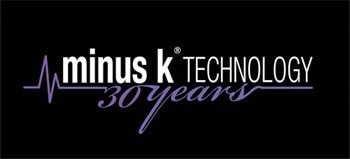
This information has been sourced, reviewed and adapted from materials provided by Minus K Technology.
For more information on this source, please visit Minus K Technology.