Sponsored by MerckJan 21 2021
Fortuitously discovered by Japanese physicist Sumio Iijima while he was studying the surface of graphite electrodes in an electric arc discharge, Carbon nanotubes (CNTs), simply known as 'nanotubes,' are cylindrical carbon allotrope nanostructures.1 Since Iijima’s revelation, CNTs have retained a key role in the field of nanotechnology due to their particular electronic, mechanical, and structural properties.1-3
CNTs possess great conductivity and high aspect ratio which enables the formation of a network of conductive tubes. Their exceptional mechanical properties derive from an amalgamation of strength, stiffness and tenacity.4 Integrated into a polymer, CNTs shift their mechanical load to the polymer matrix at a weight percentage significantly lower than those of carbon black or carbon fibers, promoting applications with greater efficiency.
CNTs have also been used for thermal protection as thermal interface materials. Their unique electronic and mechanical properties can be utilized across a diverse range of applications, such as nanocomposite materials,5 nanosensors,6 field-emission displays7, and logic elements.8
The utility of CNTs has been studied in great detail ranging from applications in pioneering electronic fabrication extending to pharmaceutical fields for treatment of many different types of disease.9
Single-Walled Carbon Nanotubes
Single-walled carbon nanotubes (SWNTs) (Product No. 755710) are smooth ordered cylinders consisting of a layer of graphene. They have exceptional electronic properties which can differ considerably with the chiral vector, C = (n, m), the parameter that specifies how the graphene sheet is rolled to produce a carbon nanotube.10
The electrical conductivity of SWNTs relies on the (n, m) values as illustrated in Table 1. Accordingly, how they are rolled directs the SWNTs' bandgap which can vary from 0 to 2 eV while electrical conductivity can demonstrate metallic or semiconducting behavior.
Table 1. The theoretical electronic conductivity of single-walled carbon nanotubes (SWNTs) depending on roll orientation of the graphene sheet (n, m).10 Source: Merck Millipore Sigma
(n, m) |
Form of SWNTs |
Electrical Conductivity |
(n, 0) |
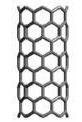
Zigzag |
Metallic when n is the multiple of 3, otherwise, semiconducting |
(n, n) |
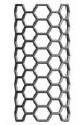
Armchair |
Metallic |
(n, m) when m ≠ 0 and n |
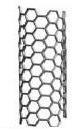
Chiral |
Metallic when (2n+m)/3 is an integer, otherwise, semiconducting |
Thermal and electrical conductivities of carbon nanotubes are exceptionally high and comparable to other conductive materials as displayed in Table 2.11
Table 2. Transport properties of carbon nanotubes and other conductive materials.11 Source: Merck Millipore Sigma
Material |
Thermal
conductivity [W/(m•K)] |
Electrical
Conductivity [S/m] |
Carbon Nanotubes |
>3,000 |
106 – 107 |
Copper |
400 |
6 x 107 |
Carbon Fiber – Pitch |
1,000 |
2 – 8.5 x 106 |
Carbon Fiber – PAN |
8 - 105 |
6.5 – 14 x 106 |
Multi-Walled Carbon Nanotubes
Multi-walled carbon nanotubes (MWNTs) (Product No. 755133) are comprised of numerous layers of graphene that have been rolled-up. Due to their structural complexity and variety in comparison to SWNTs, MWNTs have not yet been well-defined. However, MWNTs demonstrate some advantages over SWNTs, such as scalability due to simplified mass production, improved thermal and chemical stability, and low production cost per unit.
Generally, when functionalized the electrical and mechanical properties of SWNTs can fluctuate, this is due to the structural defects when C=C bond breakages occur during chemical processes. However, the innate properties of carbon nanotubes can be retained by the surface modification of MWNTs: exposure of the outer wall of MWNTs to chemical modifiers.
Surface modification of CNTs is conducted to establish new properties in carbon nanotubes for distinctive applications that necessitate enhancement of functionality, organic solvent or water-solubilization, dispersion, and compatibility or reducing the toxicity of CNTs.12 Figure 1 exhibits several ways to chemically modify the surface of CNTs.
Common functionalized CNTs, such as MWNT-COOH (Product No. 755125), are acquired via oxidation using a variety of acids, ozone, or plasma, which produces other oxygen functional groups (e.g., -OH, -C=O). The existence of oxygen-containing groups encourages the exfoliation of CNT bundles and improves the solubility in polar media and the chemical affinity with compounds containing ester, such as polyesters.
COOH groups on nanotube surfaces are beneficial sites for advanced modification. Different molecules, such as synthetic and natural polymers can be grafted through the production of amide and ester bonds.13
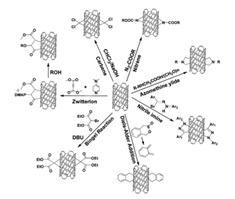
Figure 1. Schematic examples of surface functionalization of CNTs. (Illustration from Zhao et al.12)
Double-Walled Carbon Nanotubes
Double-walled carbon nanotubes (DWNTs) (Product No. 755168, 755141) are a synthetic combination of both single-walled and multi-walled nanotubes, exhibiting properties intermediate between the two types. DWNTs are made up of precisely two concentric nanotubes separated by 0.35 – 0.40 nm, with band gaps appropriate for use in field-effect transistors.14 The inner and outer walls of DWNTs have the optical and Raman scattering attributes of each wall.15
Theoretically, if each wall acts like an SWNT and according to (n, m) values of their inner and outer walls, DWNTs can hold four combinations based on the electronic type (metallic or semiconducting) e.g., metallic-metallic (inner-outer), metallic-semiconducting, semiconducting-metallic, and semiconducting-semiconducting.
Several innovative studies discovered that DWNTs may behave as a metal even though both walls are semiconducting.16 This particular snag concerning their overall electrical behavior has restricted the utility of DWNTs to applications such as thin-film electronics.
Yet, DWNTs also demonstrate a range of advantageous properties observed from MWNTs, such as enhanced lifetimes and current densities for field emission and great stability under hostile chemical, mechanical and thermal treatments along with the flexibility observed with SWNTs.17
Selective functionalization of the DWNTs outer wall has promoted their use as core-shell systems made of a clean carbon nanotube core and chemically-functionalized nanotube shells, which are appropriate as imaging and therapeutic agents in biological systems.18
DWNTs can be employed in gas sensors19 as sensitive materials for the detection of gases such as H2, O2, NO2 or NH3, dielectrics,20 and technically challenging applications, such as photovoltaics and field-emission displays.21
Merck offers premium quality SWNTs, MWNTs, and DWNTs, among which are some of the most electrically conductive additives on the market today, ready for your creative and advanced materials research needs. Where indicated, these nanotubes are developed via the catalytic chemical vapor deposition (CCVD) technique, a well-known industrial process with a proven reliability and scalability factor.
Merck’s nanotubes are also purified or functionalized to enhance the performance for research applications where particular chemical properties like high field emission characteristics, large surface area, or transparency are required.
Carbon nanotubes can be utilized across a broad range of new and existing applications including those listed below:
- Antifouling paint
- Atomic Force Microscope (AFM) tips
- Batteries with improved lifetime
- Bioengineering applications such as energy storage and conversion devices, radiation sources, and hydrogen storage media
- Biosensors for harmful gases
- Conductive plastics
- Extra strong and conductive fibers
- Flat-panel displays
- Gas storage
- High functional textiles
- Micro- and nano-electronics
- Radar-absorbing coating
- Structural composite materials
- Targeting Drug Delivery
- Ultra-capacitors
Detailed descriptions of available carbon nanotubes are exhibited in Table 3. The presented specification details will help guide you in selecting the appropriate material for your application.
Table 3. Specification details for carbon nanotubes. Source: Merck Millipore Sigma
Product No. |
TEM Image |
Description |
755710 |
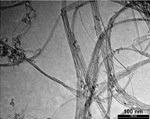 |
- Single-walled isolated and bundled carbon Nanotubes powder
- Carbon purity: >70% (by TGA)
- High specific surface area (>1000 m2/g by BET)
- 2 nm (diameter, measured by HRTEM) x several µm (length, measured by TEM / SEM)
- Metal oxide impurity: <30% (by TGA)
|
755168 |
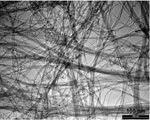 |
- Double-walled isolated and bundled carbon nanotubes powder
- High filed emission characteristics
- 3.5 nm (diameter, by HRTEM) x 1 - 10 µm (length, by TEM / SEM)
- Carbon purity: >90% (by TGA)
- Metal oxide impurity: <10% (by TGA)
- Specific surface area: >500 m2/g (by BET)
- Transparency
|
755141 |
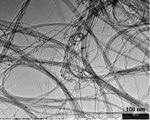 |
- Short double-walled isolated and bundled carbon nanotubes powder
- Surface chemistry characteristics
- 3.5 nm (diameter, by HRTEM) x 3 µm (length, by TEM / SEM)
- Metal oxide impurity: <10% (by TGA)
- Carbon purity: >90% (by TGA)
- Ease of dispersibility
|
755133 |
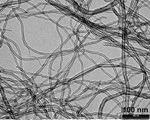 |
- Thin multi-walled (avg. 7~9 walls) carbon nanotubes powder
- 9.5 nm (diameter, by TEM) x 1.5 µm (length, by TEM)
- High level of purity
- Metal oxide impurity: <5% (by TGA)
- Carbon purity: >95% (by TGA)
|
755125 |
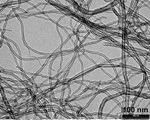 |
- Thin multi-walled (avg. 7~9 walls) -COOH functionalized carbon nanotubes powders
- 9.5 nm (diameter, by TEM) x 1.5 µm (length, by TEM)
- COOH functionalization: >8%
- Metal oxide impurity: <5% (by TGA)
- Carbon purity: >95% (by TGA)
- High level of purity
|
*TEM images with reprints permission granted by Nanocyl SA.
References and Further Reading
- S. Iijima, Nature 1991, 354, 56-58.
- X. Peng, S. S. Wong, Advanced Materials 2009, 21, 625-642.
- C. Prabhakar, K. B. Krishna, Research Journal of Pharmaceutical, Biological and Chemical Sciences 2011, 2, 850-854.
- S. Belluci, Physical Status Solidi C 2005, 2, 34-47;
H.G. Chae, S. Kumar, Journal of Applied Polymer Science 2006, 100, 791-802;
M. Meo, M. Rossi, Composites Science and Technology 2006, 66, 1597-1605.
- M. T. Byrne, Y. K. Gun’ko, Advanced Materials 2010, 22, 1672-1688.
- B. L. Allen, P. D. Kichambare, A. Star, Advanced Materials 2007, 19, 1439-1451.
- Z. Liu, L. Jiao, Y. Yao, X. Xian, J. Zhang, Advanced Materials 2010, 22, 2285-2310.
- P. Sharma, P. Ahuja, Materials Research Bulletin 2008, 43, 2517-2526.
- V. Prajapati, P. K. Sharma, A. Banik, Research Journal of Pharmaceutical, Biological and Chemical Sciences 2011, 2, 1099-1107.
- S. Ogata, Y. Shibutani, Physical Review B 2003, 68, 165409.
- List of thermal conductivities, http://en.wikipedia.org/wiki/List_of_thermal_conductivities
- H. Wu, X. Chang, L. Liu, F. Zhaoa, Y. Zhao, Journal of Materials Chemistry 2010, 20, 1036-1052.
- R. Sitko, B. Zawisza, E. Malicka, Trends in Analytical Chemistry 2012, 37, 22-31.
- K. Liu, W. Wang, Z. Xu, X. Bai, E. Wang, Y. Yao, J. Zhang, Z. Liu, Journal of American Chemical Society 2008, 131, 62-63.
- Y. Piao, C. Chen, A. A. Green, H. Kwon, M. C. Hersam, C. S. Lee, G. C. Schatz, Y. Wang, Journal of Physical Chemistry Letters 2011, 2, 1577-1582.
- Y. Tison, C. E. Giusca, V. Stolojan, Y. Hayash, S. R. P. Silva, Advanced Materials 2008, 20, 189-194.
- A. A. Green, M. C. Hersam, ACS Nano 2011, 5, 1459–1467.
- A. H. Brozena, J. Moskowitz, B. Y. Shao, S. L. Deng, H. W. Liao, K. J. Gaskell, Y. H. Wang, Journal of American Chemical Society 2010, 132, 3932-3938.
- I. Sayagoa, H. Santosa, M.C. Horrillo a, M. Aleixandrea, M. J. Fernándeza, E. Terradob, I. Tacchini, R. Arozc,W.K. Maserb, A.M. Benitob, M.T. Martínezb, J. Gutiérreza, E. Munoz, Talanta, 2008, 77, 758-764.
- L. G. Moura, C. Fantini, A. Righi, C. Zhao, H. Shinohara, M. A. Pimenta, Physical Review B 2011, 83, 245427.
- A. C. Dillon, Chemical Reviews 2010, 110, 6856-6872.
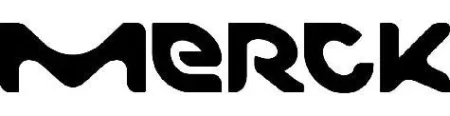
This information has been sourced, reviewed and adapted from materials provided by Merck.
For more information on this source, please visit Merck.