Sponsored by MerckReviewed by Emily MageeJan 23 2024
Fluorescent nanodiamonds (FNDs) possess unique characteristics, including high biological compatibility, infinite photostability, the absence of photoblinking, a long fluorescence lifetime (>10 ns), and ease of biofunctionalization.
These features make FNDs an appealing alternative for imaging reagents compared to quantum dots, organic dyes, and fluorescent polymer beads.1-3
The fluorescence in FNDs originates from nitrogen-based color centers. Their infinite photostability stems from the fact that these color centers are incorporated into the lattice structure and are well-protected from the surrounding environment,
Applications of FNDs encompass background-free long-term cell imaging, cell progeny tracing, flow cytometry, super-resolution imaging, correlative microscopy, labeling of low-abundance cellular components, and image-guided surgery.3-4
In addition to its distinct fluorescence, the negatively charged nitrogen-vacancy color center within FND (Fluorescent Nanodiamond) exhibits spin activity. This allows for fluorescence control in response to external influences like electromagnetic fields, temperature, and strain.
Such traits make FND an exceptional material for quantum nanosensors, facilitating precise measurements of temperature changes within cells.5
Characteristics of Fluorescent Nanodiamonds: Color, Brightness, and Size
Light emission from primary color centers in FNDs spans the whole visible spectrum, with the emission wavelength being altered by adjusting the nitrogen (N) and vacancies (V) substitutions.
For instance, an FND with NV centers emits red or near-infrared light, while NVN centers emit green light. FNDs featuring N3 centers emit blue light. Among these color centers, NV and NVN centers have been the most widely studied.
The intensity of fluorescence in FNDs increases with particle size, which ranges between 10 nm to 1 μm. Larger particle volumes provide more centers per particle, boosting fluorescence intensity.
An 80 nm FND with 3 ppm NV- centers demonstrates about 10 times brighter emission intensity compared to a single Atto 532 dye molecule, as measured by electron paramagnetic resonance.6 As a carbon-based material, FND surface modification relies on altering organic functional groups.
Key chemical functionalities for FNDs include carboxylic acids (-COOH), hydroxyls (-OH) groups, and amines (-NH2).
These functionalities allow the attachment of ligands using various standard organic chemistries, such as amidation, esterification, and silanization. Carboxylated FNDs serve as a versatile starting point for subsequent modifications essential for biological use.
Nanodiamonds strongly scatter light, useful for guiding or weakening light. Visually, solutions with larger nanodiamond particles appear milky-white until roughly 50 nm. Below 50 nm, the solution gradually becomes transparent brown/amber (Figure 1).
The clarity of transmitted light indicates particle size, aiding in monitoring colloidal stability during particle functionalization. Haze or grayness after functionalization or alteration of nanodiamonds, when viewed sideways, may suggest aggregation.
In many cases, this aggregation is reversible and can be resolved by resuspending the particles in a solvent better suited to the particle's surface chemistry (hydrophilic or hydrophobic).
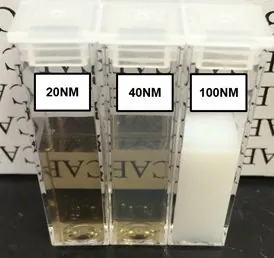
Figure 1. Suspensions of 20, 40, and 100 nm FND particles in deionized water. Extensive light scattering of large particles causes the 100 nm suspension to appear milky-white in color. The slight reduction in optical clarity of the sample containing 40 nm particles as compared to the one with 20 nm particles is indicative of greater light scattering from larger particles. Suspensions adopt a brown/amber color when sizes are small enough that light scattering is reduced. In all these suspensions the concentration of FNDs is approximately 1 mg/mL. Image Credit: Merck
Although commercial FND particles are mostly sold based on weight per unit volume (w/v), knowing their particle number concentration (that is, particles per unit volume) is crucial for further surface modification. As particle size decreases under constant mass loading, the particle number concentration drastically rises.
This boosts the number of potential reactive sites and decreases inter-particle spacing, thus elevating the probability of aggregation. Hence, successful functionalization demands adjustment of the particle number concentration.
The particle number concentration (C (M)) can be easily calculated by inputting the particle diameter d (nm) and weight/volume concentration (x in mg/mL) derived from diamond density, mass per particle, and Avogadro’s constant.
 |
1 |
The number of available surface sites in a reaction (N) depends on the surface COOH groups' areal density (ρ, approximately 0.5-1 group/nm2), volume of particle solution used (V), and particle surface area (d):
 |
2 |
Biofunctionalization Strategies for Fluorescent Nanodiamonds
To attach molecules to ND surfaces, the carbodiimide coupling of amines to carboxylic acids stands out as an effective method. EDC/NHS [1-ethyl-3-(3-dimethylaminopropyl) carbodiimide/N-hydroxysuccinimide], a commonly used variant, facilitates the direct reaction of primary amine-terminated groups with carboxylic acids.
An alternative approach involves an intermediate activation step, where the carboxylic acid is first activated by NHS or NHS-sulfo, followed by the reaction with amines after cleaning the coupling reagents.7
This second route proves particularly beneficial for hetero-functional groups containing both carboxylic acids and amines (like proteins) that might undergo polymerization. Successful functionalizations often decrease inter-particle electrostatic repulsions, which contribute to the stability of particles covered with ionogenic groups dispersed in a solvent.
Since both EDC and NHS passivate surface negative charges, the reduced zeta potential decreases the stabilizing repulsions, potentially resulting in aggregation.
NHS-sulfo has a conjugated sulfate group. It is commonly used to enhance particle stability during functionalization by maintaining a negative charge.
To counter the adverse effects in biological media caused by elevated ionic strength, functionalization with hyperbranched PEGs has proven effective.8
Other strategies to maintain particle stability include the use of PEGylated carbodiimides9 or a reduction in the total concentration of reagents and particles. Another facile method for linking biomolecules to the diamond surface is through click chemistry-mediated conjugation.
For instance, tetrazine-functionalized ND can be reacted with transcyclooctene at room temperature to label particles with antibodies or proteins with rapid reaction kinetics (up to 26000 M-1s-1).10-12
This conjugation method benefits from the removal of a reaction catalyst, high orthogonality, and a reduction in expensive reagent waste.13
Protein Modified Fluorescent Nanodiamonds
The avidin-biotin interaction stands out as one of the most popular strategies in biotechnological and biomedical applications, particularly for intracellular labeling with FNDs.2 Any form of ND modification is best supported by PEGylation, which offers a soft layer that preserves the protein form and reduces steric hindrance.
Large, submicron-sized FNDs, which can observed individually in a regular fluorescent microscope, are useful for validating reaction efficiency and serving as a platform for chemical developments and educational outreach.
Biotinylated FNDs with NVN centers (green) and streptavidin functionalized FNDs with NV centers (red)—each carrying multiple copies of either streptavidin or biotin—were utilized for the experimental results in Figure 2.
Incubation time and particle concentrations significantly influence the resulting conjugates. Low concentrations of starting red and green FNDs with short incubation times result in dimers and trimers of conjugated particles (as shown in Figure 2a).
Over time, due to the multivalency of the particles, multi-particle aggregates assemble in an ordered manner with alternating colors (as shown in Figure 2b). This alternate arrangement of streptavidin and biotin-functionalized FNDs suggests the potential use of these particles for particle self-assembly.5
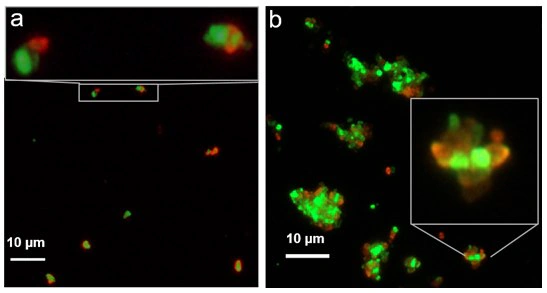
Figure 2. Images of coupled sub-micron biotinylated FNDs containing NVN centers (green) and streptavidin functionalized FNDs containing NV centers (red), comparing a) 30-minute incubation time and b) overnight incubation. Image Credit: Merck
Using the same streptavidin chemistries shown earlier, the labeling of a biotin-linked internal target in mouse macrophage cells with 40 nm streptavidin functionalized red FNDs illustrates that the brightness of 40 nm FNDs is sufficient for intracellular labeling.
Conjugation Protocols for Nanodiamonds
a) Streptavidin Functionalization
Carboxy polyethylene glycol ND (ND-PEG-COOH) serves as an excellent starting point for streptavidin functionalization. While the reaction can occur in water, using DMF boosts ND stability, enhances NHS-sulfo activation, and minimizes aggregation.
It is crucial to account for the number of available reactive sites when determining specific reaction conditions—as this changes with size.
- Centrifuge ND particles in water at the lowest RCF needed for pellet formation.
- Suspend the pellet in DMF to <50 nM (typically <1 mg/mL) and sonicate in a water bath for five minutes for easy resuspension.
- Introduce NHS-sulfo and EDC in roughly 300 times molar excess compared to the reactive sites, starting with NHS-sulfo. For a 50 nm particle, an estimated amount of 140 nmol/mg for NHS-sulfo and EDC can be calculated using expressions 1 & 2. 1 mg of EDC/NHS is generally sufficient unless aggregation arises, then reduce the amount of EDC/NHS.
- Briefly vortex the solution from step 3, and let it react for 30 minutes with intermittent vortexing and bath sonication. The solution might turn slightly hazy with mild aggregation.
- Wash the particles with DMF by pelleting at a low RCF (<1000 x g should suffice) and resuspending in DMF.
- Pellet at a low RCF, resuspend in water (<50 nM, typically <1 mg/mL), and sonicate to fully break the pellet. For maximum stability, perform conjugation in DI or low ionic strength (<10 mM) buffer at this stage.
- Add an excess of streptavidin. Adjust streptavidin quantity based on desired coverage, allowing the reaction to occur for two to four hours (100x streptavidin compared to particle number concentration is a good starting point). Reduce if aggregation is observed; excess prevents interparticle bridging by streptavidin.
- Wash particles at least twice with the preferred end buffer to remove excess streptavidin. If centrifugation is used, quench the remaining reactive groups on the ND surface by adding a small molecule containing an amine (tris or glycine) to prevent aggregation from pelleting. Alternatively, use dialysis with a large pore size (>150 kDa MWCO) membrane to avoid aggregation due to centrifugation. For increased stability, resuspend final particles in 0.1 % BSA in PBS.
- Store functionalized FNDs with streptavidin at 4 °C (avoid freezing). Properly stored functionalized NDs should maintain activity for several months.
b) Biotinylation
Biotinylation of NDs is easily achieved by directly reacting NHS-conjugated PEG-biotin (Aldrich Prod. No. 670049) with aminated NDs (ND-NH2). Alternatively, aminated NDs with a PEG coating can be directly conjugated to Biotin-NHS.
- ND-NH2 is separated by low RCF centrifugation (e.g., 5000 rcf) from water and then re-suspended in DMF (or an alternative NHS conjugation buffer).
- NHS-PEG-Biotin is added in the desired molar excess to reactive sites (1-10x) and left to react overnight.
- Particles are washed twice with DMF or water and suspended in the desired final buffer.
- Store particles at 4 °C (avoid freezing). Properly stored functionalized NDs should remain active for several months.
c) Coupling Biotinylated/streptavidin Functionalized Nanodiamonds
The coupling of biotinylated and streptavidin-functionalized components is straightforward, involving a brief incubation of the two for short periods (e.g., 30 minutes).
Coupling larger particles or inter-particle coupling may require a longer conjugation time due to reduced translational and rotational freedom induced by particle size and statistical binding chances.
When designing incubation times, it is essential to consider and adjust the multivalency of either streptavidin or biotinylated elements. Blocking agents (such as BSA, detergent, and glycerol) can be introduced to prevent nonspecific binding without compromising streptavidin/biotin activity.
In summary, FNDs serve as excellent platforms for biomedical applications requiring prolonged and/or intense illumination. Conjugating FNDs is akin to typical organic-based molecular fluorochromes, and their per-particle brightness proves highly useful for labeling low-level expressed targets.
Integrating them into the laboratory toolkit expands researchers' capabilities beyond traditional organic dyes.
Request the "Nanomaterial Bioconjugation Techniques" guide for step-by-step protocols on surface modification and bioconjugation of inorganic nanomaterials for diagnostic applications.
Acknowledgment: This work received funding from the NHLBI, Department of Health and Human Services, under Contract No. HHSN268201500010C.
References and Further Reading
- Mochalin VN, Shenderova O, Ho D, Gogotsi Y. 2012. The properties and applications of nanodiamonds. Nature Nanotech. 7(1):11-23. https://doi.org/10.1038/nnano.2011.209
- Reineck P, Francis A, Orth A, Lau DWM, Nixon?Luke RDV, Rastogi ID, Razali WAW, Cordina NM, Parker LM, Sreenivasan VKA, et al. 2016. Brightness and Photostability of Emerging Red and Near?IR Fluorescent Nanomaterials for Bioimaging. Advanced Optical Materials. 4(10):1549-1557. https://doi.org/10.1002/adom.201600212
- Vaijayanthimala V, Lee DK, Kim SV, Yen A, Tsai N, Ho D, Chang H, Shenderova O. 2015. Nanodiamond-mediated drug delivery and imaging: challenges and opportunities. Expert Opinion on Drug Delivery. 12(5):735-749. https://doi.org/10.1517/17425247.2015.992412
- Chang B, Lin H, Su L, Lin W, Lin R, Tzeng Y, Lee RT, Lee YC, Yu AL, Chang H. 2013. Highly Fluorescent Nanodiamonds Protein-Functionalized for Cell Labeling and Targeting. Adv. Funct. Mater.. 23(46):5737-5745. https://doi.org/10.1002/adfm.201301075
- Wu Y, Jelezko F, Plenio MB, Weil T. 2016. Diamond Quantum Devices in Biology. Angew. Chem. Int. Ed.. 55(23):6586-6598. https://doi.org/10.1002/anie.201506556
- Shenderova O, Nunn N, Oeckinghaus T, Torelli M, McGuire G, Smith K, Danilov E, Reuter R, Wrachtrup J, Shames A, et al. 2017. Commercial quantities of ultrasmall fluorescent nanodiamonds containing color centers. https://doi.org/10.1117/12.2256800
- 2013. Hermanson, G. T. Bioconjugate techniques. Academic press..
- Boudou J, David M, Joshi V, Eidi H, Curmi PA. 2013. Hyperbranched polyglycerol modified fluorescent nanodiamond for biomedical research. Diamond and Related Materials. 38131-138. https://doi.org/10.1016/j.diamond.2013.06.019
- Shen H, Jawaid AM, Snee PT. 2009. Poly(ethylene glycol) Carbodiimide Coupling Reagents for the Biological and Chemical Functionalization of Water-Soluble Nanoparticles. ACS Nano. 3(4):915-923. https://doi.org/10.1021/nn800870r
- Blackman ML, Royzen M, Fox JM. 2008. Tetrazine Ligation: Fast Bioconjugation Based on Inverse-Electron-Demand Diels?Alder Reactivity. J. Am. Chem. Soc.. 130(41):13518-13519. https://doi.org/10.1021/ja8053805
- Knall A, Slugovc C. 2013. Inverse electron demand Diels?Alder (iEDDA)-initiated conjugation: a (high) potential click chemistry scheme. Chem. Soc. Rev.. 42(12):5131. https://doi.org/10.1039/c3cs60049a
- Devaraj NK, Weissleder R. 2011. Biomedical Applications of Tetrazine Cycloadditions. Acc. Chem. Res.. 44(9):816-827. https://doi.org/10.1021/ar200037t
- Escorihuela J, Marcelis ATM, Zuilhof H. 2015. Metal?Free Click Chemistry Reactions on Surfaces. Adv. Mater. Interfaces. 2(13):1500135. https://doi.org/10.1002/admi.201500135
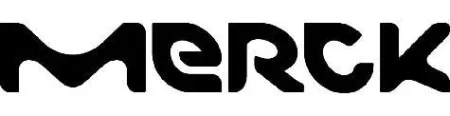
This information has been sourced, reviewed, and adapted from materials provided by Merck.
For more information on this source, please visit Merck.