Sponsored by MerckReviewed by Emily MageeJan 23 2024
Over the recent decades, the use of gold nanoparticles in research and various industries has significantly grown, spanning from biomedical engineering1 to photovoltaics.2
The optical qualities of these particles, which can be adjusted by altering their shape and size, are widely exploited in numerous applications.
Uncoated gold nanoparticles are prone to aggregation in solution and melting when exposed to laser irradiation, leading to notable alterations in their optical traits.
Once their surfaces are appropriately passivated by chemical functionalization, these gold nanoparticles can resist clumping and shape distortion across different biological, physical, and environmental conditions, thereby maintaining their optical characteristics.
Silica coating is a robust functionalization that has enhanced both the thermodynamic and chemical stability of gold nanoparticles,1,3,4 making it a preferred choice for various applications as described below. Examples of silica-coated gold nanospheres and nanorods are depicted in Figure 1.
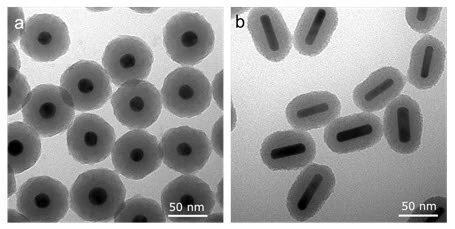
Figure 1. Scanning electron microscopy (SEM) image of (a) Silica-coated gold nanospheres and (b) silica-coated gold nanorods. Image Credit: Merck
Surface Chemistry and Synthesis of Silica-Coated Gold
Silica coating of gold nanoparticles is achieved via the Stöber method, a traditional technique that utilizes tetraethyl orthosilicate (TEOS) to generate a mesoporous, highly branched siloxane polymer on the gold’s surface.
By manipulating reaction time and reagent concentrations, the thickness of the silica layer on the gold surface can be controlled. The resulting siloxane polymer (named silica) carries hydroxyl (-OH) groups that serve as attachment points for further functionalization.
Additionally, heterobifunctional silane linkers readily interact with silica, offering a means to affix various ligands—such as polyethylene glycol (PEG)—onto the silica surface (as shown in Figure 2).
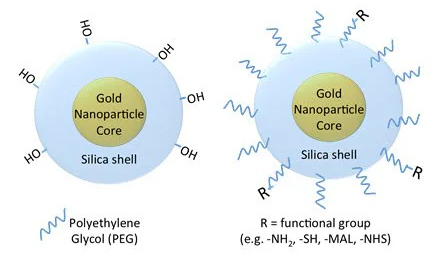
Figure 2. Silica-coated gold nanoparticle surface chemistry, showing a bare silica surface (left) with hydroxyl functional groups and a polyethylene glycol (PEG) coated nanoparticle that can be functionalized with different end groups including amines, thiols, maleimides, and N-hydroxysuccinimides, etc. Image Credit: Merck
Properties and Benefits of Silica-Coated Gold Nanoparticles
Silica coating of gold is beneficial in various applications encompassing gold nanoparticles. When utilized in applications alongside pulsed lasers, silica coating significantly improves the stability of gold nanorods.
Gold nanorods with conventional coatings, like PEG, cetyltrimethylammonium bromide (CTAB), or other small polymers, can absorb sufficient energy from pulsed lasers to undergo melting (Figure 3). This alteration in shape causes a shift in their light absorption and scattering from near-infrared (NIR) wavelengths to the visible spectrum.5
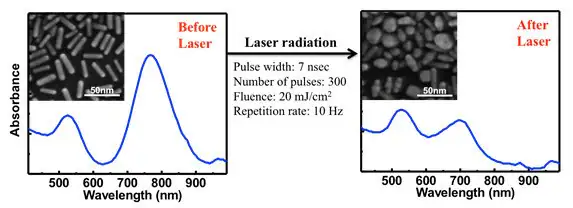
Figure 3. Gold nanorods and optical properties are shown before (left) and after pulsed laser exposure (right). Standard gold nanorods without silica coating are highly unstable and will melt in response to laser light absorption, degrading their absorption and scattering of light at near-infrared wavelengths. Image Credit: Merck
For applications reliant on consistent NIR light absorption, these regularly coated gold nanorods may yield unreliable outcomes. On the contrary, silica-coated gold nanorods resist shape alterations and can uphold their optical characteristics, even under much higher light intensity (fluence) levels (Figure 4).
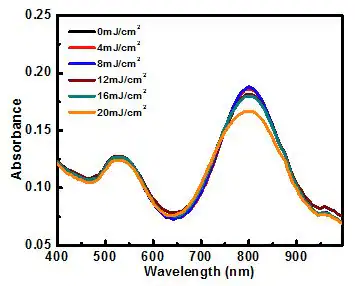
Figure 4. Absorbance spectrum of silica-coated gold nanorods after exposure to 300 pulses of 808 nm light at various levels of intensity (fluence). Silica-coated particles are thermodynamically stable (they resist shape change) for fluences up to 20 mJ/cm2. Image Credit: Merck
As well as thermodynamic and colloidal stability, the silica coating of gold nanorods provides other significant properties and advantages. For example, silica coating augments the surface area for the conjugation of antibodies or other targeting elements.
The porous nature of silica enables the loading of drugs, dye molecules, or other imaging agents, either through physical adsorption or covalent attachment.
This coating also restricts how closely nanorods can aggregate at high concentrations, minimizing the impact of plasmon coupling and ensuring the preservation of their optical properties, irrespective of concentration.
Finally, the silica coating on gold nanoparticles substantially enhances imaging contrast, such as in photoacoustic imaging, by over three times.1
Silica-coated gold nanoparticles that are PEGylated (Figure 2 - right) can provide all the advantages of silica coating along with the characteristics associated with PEG-coated nanoparticles—such as heightened colloidal stability and reduced immunogenicity.
Applications for Silica-Coated Gold Nanostructures
1. Photoacoustic Imaging
When employed as contrast agents in photoacoustic imaging, gold nanorods absorb light from a pulsed laser and produce significant heat. While this heat is necessary for the photoacoustic effect, it can reach detrimental levels that cause the nanorods to melt (Figure 2).
The alteration in shape causes a decrease in absorption cross-section, resulting in a loss of contrast in photoacoustic imaging.
Silica coating aids in reducing the interfacial thermal resistance between gold and the surrounding solvent, enabling the particle to release more heat to its surroundings. This has two positive effects.
Firstly, gold particles can withstand high fluences without melting (Figure 3). Secondly, the photoacoustic signals created from these particles are at least three times greater than those from gold nanoparticles with standard coatings like PEG, CTAB, or other small polymers.1
Thermodynamically stable, silica-coated gold nanorods and nanospheres have gained popularity as methods for photoacoustic contrast and therapeutic agents, due to their superior stability, optical properties, biocompatibility, and potential for bioconjugation.1
2. Cell Tracking
Photoacoustic imaging (optoacoustic imaging) is a noninvasive, quantitative method with brief scan times. It is therefore an ideal tool for tracking immune cells8 and monitoring stem cell implants10 coupled with ultrasound imaging.
The utilization of silica-coated gold nanorods as contrast agents in photoacoustic imaging allows for real-time quantification of implanted cells and verification of their arrival at the treatment site. The silica coating also aids in enhancing the cellular uptake of the gold nanorods.8
Silica-coated gold nanorods are extensively applied in photothermal therapy due to their small size, adjustable resonance in the red and NIR spectrum, and notably high absorption cross-section.
The silica coating improves the photothermal stability of gold nanorods, ensuring that they retain superior optical properties (even under high laser fluencies).1,3,6
Compared to PEG-coated gold nanorods, silica-coated gold nanorods exhibit significantly increased cellular uptake, thereby resulting in a more effective photothermal ablation effect.10
Coupled with photoacoustic imaging, silica-coated nanorods enable the creation of heat generation maps during photothermal therapy that can support its dosage and therapeutic effectiveness.7
3. Targeted Drug Delivery
Biocompatible silica-coated gold nanoparticles can be chemically altered for precise targeting of cancerous tissues.
Mesoporous silica-coated gold nanoparticles act as carriers for anticancer drugs like doxorubicin, DNA, and proteins due to their large surface area, adjustable size, highly accessible pore volume, drug loading capacity, and well-defined surface properties that permit chemical modification (as shown in Figure 4).
Chemo–photothermal therapy (achieved via drug-loaded silica-coated gold nanorods) exhibits a stronger anti-cancer effect compared to both chemotherapy and photothermal therapy alone.11
4. Multiplex Imaging
Targeted silica-coated gold nanorods function as contrast agents in multiplex imaging, distinguishing cell inclusions in vitro by selectively binding to the nanoparticles expressing different receptors.12
By employing silica-coated gold nanorods with distinct peak wavelengths, each unique cell type can be labeled, allowing the identification of specific cell locations and the generation of corresponding molecular expression images.
5. Dual Mode/Multimodal Imaging
Optical imaging techniques, while highly sensitive with superior tissue resolution, face limitations due to low light penetration in tissues. Combining complementary imaging methods optimizes both sensitivity and specificity.
For example, a contrast agent that enhances both CT contrast and NIR optical imaging can deliver quantifiable information regarding contrast agent accumulation at various levels.
Mesoporous silica-coated gold nanorods loaded with an organic NIR dye, like Indocyanine Green (ICG), serve as imaging probes for dual-mode X-Ray CT and NIR fluorescence imaging.13
6. Surface Enhanced Raman Spectroscopy (SERS)
Silica-coated gold nanoparticles embedded with dye show strong effectiveness in enhancing surface Raman signals. They serve as spectroscopic tags for diverse detection and spectroscopy.14
These particles consist of a metallic core for optical signal enhancement, an encapsulating silica shell for safety and conjugation, and a reporter molecule for spectroscopic signature.
Silica-coated gold nanorods also function as dual-mode imaging probes for targeting tumor cells through both fluorescence and enhanced Raman scattering. By using distinct nanoparticle excitation wavelengths, these signals can be visualized separately.17
7. Two-Photon Imaging
Gold nanorods coated with mesoporous silica, integrated with photosensitizers like Pd-meso-tetra (4-carboxyphenyl) porphyrin (PdTPPs), can be utilized for two-photon-activated photodynamic therapy.16
The photosensitizer is doped the nano-channels of the mesoporous silica shell. It can be stimulated by intra-particle plasmonic resonance energy transfer from the enclosed two-photon excited gold nanorod and can produce harmful singlet oxygen to destroy cancer cells.
The robust silica matrix enhances the stability of the gold nanorod's two-photon luminescence by providing mechanical support against thermal deformation.
8. Biomolecular Probes
Silica-coated gold nanoparticles serve as markers for DNA hybridization assays. They detect lower concentrations of target DNA compared to citrate-coated or standard gold nanoparticles—due to the high emissivity of silica in the mid-infrared range.
Using silica-coated gold nanoparticles as robust labels that do not degrade, photobleach, or quench,17 enhances fluorescence-based DNA hybridization and protein binding assays.
This nanoparticle platform can identify minute quantities of proteins, pesticides, bacteria, and small molecules like mercury.
9. Catalysts
To function effectively as catalysts, gold nanoparticles need robust stability in heat and the surrounding medium while retaining their catalytic activity during recycling.
Gold nanorods usually get coated with the surfactant CTAB. In organic solutions, the desorption of CTAB into the medium can lead to gold nanorod aggregation and loss of their catalytic abilities.
CTAB-coated gold nanorods can also become thermodynamically unstable and transform into spherical particles. Mesoporous silica-coated gold nanorods prove to be highly efficient catalysts because their silica shell offers substantial stability against adverse conditions like heat, solvent exchange, and centrifugation.18
Since mesoporous silica shells boast large pore volumes, reactant molecules can move through the pores and reach the gold surface for catalysis.
10. Photonics
Plasmonic gold nanoparticles find utility in various photonics applications, such as single molecule detection, building photonic crystals, and designing optical devices like waveguides. However, the physical contact between the metal of adjacent bare gold nanoparticles hinders the formation of photonic band gaps.
Coating the gold nanoparticles with an optically transparent, chemically inert, and photochemically stable material—like silica—enables the formation of complete photonic band gaps when these plasmonic nanoparticles are arranged periodically.19
The silica coating also aids in enhancing plasmonic fluorescence by preventing the quenching mechanism that occurs when fluorophores directly bind to a metallic surface. Hence, silica-coated gold nanoparticles offer multiple advantages compared to standard gold nanoparticles.
In summary, the silica coating of gold nanoparticles offers several beneficial properties:
- Enhanced imaging signal strength in photoacoustic imaging and other modalities using pulsed lasers.
- Improved thermal and colloidal stability.
- Stable optical characteristics that are suitable for a broader range of applications, such as multimodal imaging.
- Theranostic capability, facilitating use as a hybrid drug carrier and imaging agent.
- Flexible silane chemistry for covalent functionalization and conjugation.
These distinct advantages position them ideally for the numerous applications highlighted in this article.
References and Further Reading
- Chen Y, Frey W, Kim S, Kruizinga P, Homan K, Emelianov S. 2011. Silica-Coated Gold Nanorods as Photoacoustic Signal Nanoamplifiers. Nano Lett.. 11(2):348-354. https://doi.org/10.1021/nl1042006
- Yang J, You J, Chen C, Hsu W, Tan H, Zhang XW, Hong Z, Yang Y. 2011. Plasmonic Polymer Tandem Solar Cell. ACS Nano. 5(8):6210-6217. https://doi.org/10.1021/nn202144b
- Chen Y, Frey W, Kim S, Homan K, Kruizinga P, Sokolov K, Emelianov S. 2010. Enhanced thermal stability of silica-coated gold nanorods for photoacoustic imaging and image-guided therapy. Opt. Express. 18(9):8867. https://doi.org/10.1364/oe.18.008867
- Luke GP, Bashyam A, Homan KA, Makhija S, Chen Y, Emelianov SY. 2013. Silica-coated gold nanoplates as stable photoacoustic contrast agents for sentinel lymph node imaging. Nanotechnology. 24(45):455101. https://doi.org/10.1088/0957-4484/24/45/455101
- Zijlstra P, Chon JWM, Gu M. 2009. Five-dimensional optical recording mediated by surface plasmons in gold nanorods. Nature. 459(7245):410-413. https://doi.org/10.1038/nature08053
- Kim S, Chen Y, Luke GP, Emelianov SY. 2014. In-vivo ultrasound and photoacoustic image-guided photothermal cancer therapy using silica-coated gold nanorods. IEEE Trans. Ultrason., Ferroelect., Freq. Contr.. 61(5):891-897. https://doi.org/10.1109/tuffc.2014.2980
- Chen Y, Frey W, Walker C, Aglyamov S, Emelianov S. 2013. Sensitivity enhanced nanothermal sensors for photoacoustic temperature mapping. J. Biophoton.. 6(6-7):534-542. https://doi.org/10.1002/jbio.201200219
- Joshi PP, Yoon SJ, Chen Y, Emelianov S, Sokolov KV. 2013. Development and optimization of near-IR contrast agents for immune cell tracking. Biomed. Opt. Express. 4(11):2609. https://doi.org/10.1364/boe.4.002609
- Jokerst JV, Thangaraj M, Kempen PJ, Sinclair R, Gambhir SS. 2012. Photoacoustic Imaging of Mesenchymal Stem Cells in Living Mice via Silica-Coated Gold Nanorods. ACS Nano. 6(7):5920-5930. https://doi.org/10.1021/nn302042y
- Zhu X, Fang C, Jia H, Huang Y, Cheng CHK, Ko C, Chen Z, Wang J, Wang YJ. Cellular uptake behaviour, photothermal therapy performance, and cytotoxicity of gold nanorods with various coatings. Nanoscale. 6(19):11462-11472. https://doi.org/10.1039/c4nr03865g
- Monem AS, Elbialy N, Mohamed N. 2014. Mesoporous silica coated gold nanorods loaded doxorubicin for combined chemo?photothermal therapy. International Journal of Pharmaceutics. 470(1-2):1-7. https://doi.org/10.1016/j.ijpharm.2014.04.067
- Bayer CL, Chen Y, Kim S, Mallidi S, Sokolov K, Emelianov S. 2011. Multiplex photoacoustic molecular imaging using targeted silica-coated gold nanorods. Biomed. Opt. Express. 2(7):1828. https://doi.org/10.1364/boe.2.001828
- Luo T, Huang P, Gao G, Shen G, Fu S, Cui D, Zhou C, Ren Q. 2011. Mesoporous silica-coated gold nanorods with embedded indocyanine green for dual mode X-ray CT and NIR fluorescence imaging. Opt. Express. 19(18):17030. https://doi.org/10.1364/oe.19.017030
- Doering WE, Nie S. 2003. Spectroscopic Tags Using Dye-Embedded Nanoparticles and Surface-Enhanced Raman Scattering. Anal. Chem.. 75(22):6171-6176. https://doi.org/10.1021/ac034672u
- Wang Z, Zong S, Yang J, Li J, Cui Y. 2011. Dual-mode probe based on mesoporous silica coated gold nanorods for targeting cancer cells. Biosensors and Bioelectronics. 26(6):2883-2889. https://doi.org/10.1016/j.bios.2010.11.032
- Chen N, Tang K, Chung M, Cheng S, Huang C, Chu C, Chou P, Souris JS, Chen C, Mou C, et al. 2014. Enhanced Plasmonic Resonance Energy Transfer in Mesoporous Silica-Encased Gold Nanorod for Two-Photon-Activated Photodynamic Therapy. Theranostics. 4(8):798-807. https://doi.org/10.7150/thno.8934
- Xia F, Zuo X, Yang R, Xiao Y, Kang D, Vallee-Belisle A, Gong X, Yuen JD, Hsu BBY, Heeger AJ, et al. 2010. Colorimetric detection of DNA, small molecules, proteins, and ions using unmodified gold nanoparticles and conjugated polyelectrolytes. Proceedings of the National Academy of Sciences. 107(24):10837-10841. https://doi.org/10.1073/pnas.1005632107
- Son M, Lee J, Jang D. 2014. Light-treated silica-coated gold nanorods having highly enhanced catalytic performances and reusability. Journal of Molecular Catalysis A: Chemical. 38538-45. https://doi.org/10.1016/j.molcata.2014.01.010
- Rodríguez-Fernández J, Pastoriza-Santos I, Pérez-Juste J, García de Abajo FJ, Liz-Marzán LM. 2007. The Effect of Silica Coating on the Optical Response of Sub-micrometer Gold Spheres. J. Phys. Chem. C. 111(36):13361-13366. https://doi.org/10.1021/jp073853n
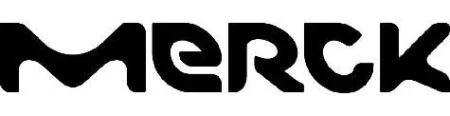
This information has been sourced, reviewed, and adapted from materials provided by Merck.
For more information on this source, please visit Merck.