Sponsored by Genizer LLCReviewed by Aimee MolineuxNov 12 2024
When developing new pharmaceuticals, many challenges are faceted. These include evading the immune system, the risk of cytotoxicity, and getting US Food and Drug Administration (FDA) approval.
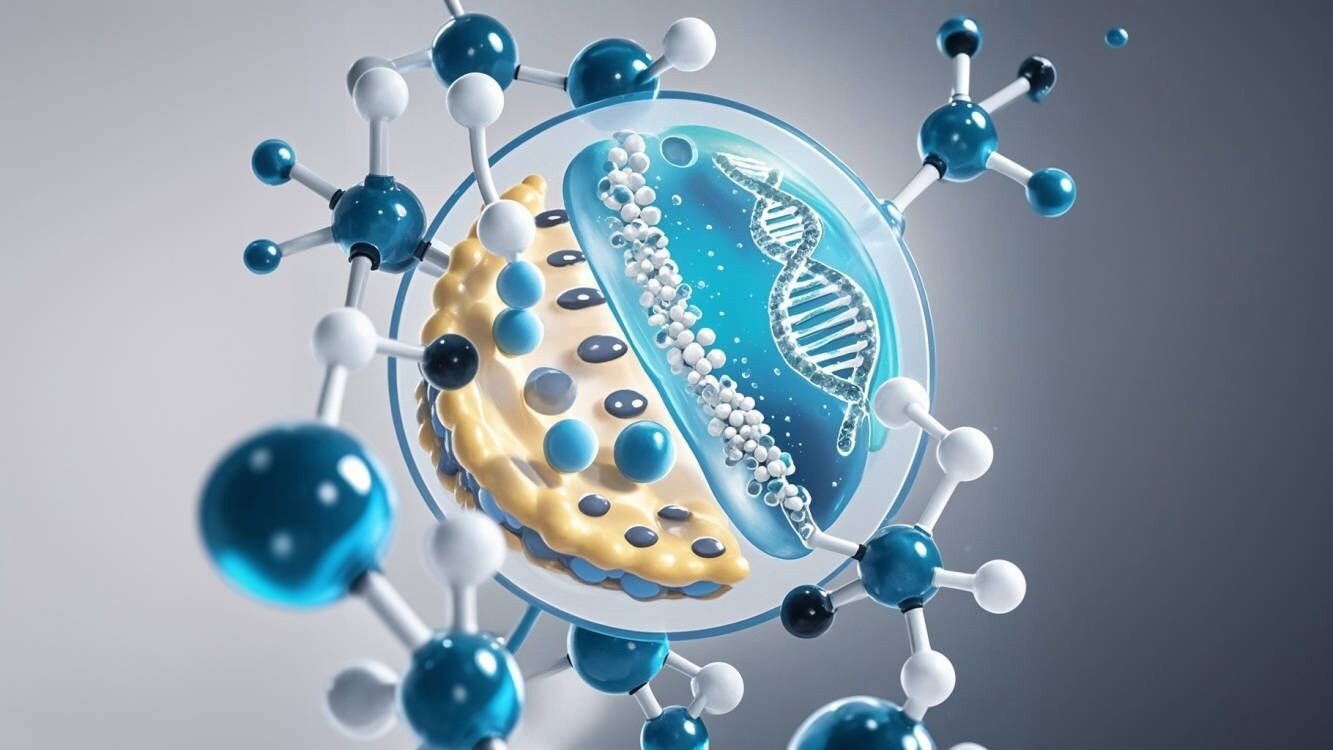
Image Credit: Genizer LLC
Overcoming these challenges relies upon proven developmental and analytical methods, steps to reduce patient harm, and techniques to extend the blood circulation time.
Encapsulating an active ingredient in a liposome or lipid nanoparticle can reduce its cytotoxicity and extend blood circulation by evading the immune system.
Several techniques can enhance a medicine’s effectiveness, including enhanced permeability and retention, active targeting, and external stimuli targeting. These techniques work by targeting a specific area. Liposomes also boast a decades-long history of approval and use as drugs in Europe and the U.S.
Lipid nanoparticles and liposomes were the first nanomedicine but remain relevant today. Using liposomes in clinical trials has increased in recent years;1 for example, the pioneering Moderna mRNA COVID-19 vaccine is a lipid nanoparticle.2
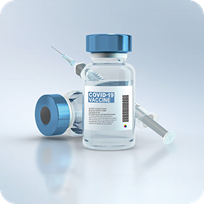
Image Credit: Genizer LLC
Liposomes
Human cell membranes are made up of phospholipid bilayers. These membranes’ insides and outsides feature hydrophilic ends, while their centers comprise hydrophobic tails.3
The phospholipid bilayer is semi-permeable. The hydrophilic center prevents hydrophobic molecules from entering the cell, though some hydrophilic molecules can pass through this.4
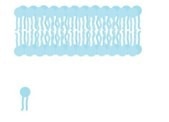
Image Credit: Genizer LLC
A liposome is a synthetic cell membrane comprised of cholesterols and phospholipids. Liposomes are employed in various applications, including cosmetics, agriculture, and pharmaceuticals.3
Because liposomes are like human cell membranes, they are biologically inert and biodegradable.5
The combination of hydrophilic and hydrophobic parts can prompt the natural formation of spherical liposomes—the hydrophilic ends are drawn to one another, and the hydrophobic heads are also drawn to one another.5,6 Researchers can also resize the resulting liposomes to suit their needs.
Liposome size ranges from 0.025 to 2.5 μm in diameter,5 though biomedical researchers utilize liposomes in the 50 to 450 nm range.7 Liposomes’ properties can differ based on their formulation, making them extremely versatile.3
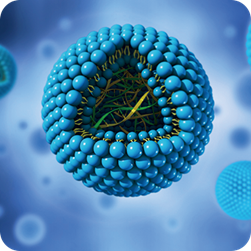
Image Credit: Genizer LLC
While liposomes represent one of the earliest forms of lipid nanoparticle,8 researchers continue to develop the next generation of lipid nanoparticles, including solid lipid nanoparticles like cationic lipid-nucleic acid complexes, which see current use in the delivery of nucleic acid drugs. The complex reduces degradation, helping to deliver drugs more effectively.8
Encapsulating an active ingredient in a liposome or other lipid nanoparticle can have various benefits, one of the most important being reducing cytotoxicity.
Biocompatibility
Cytotoxicity damages patient cells, causing side effects to worsen and limiting patient compliance. Encapsulating an active ingredient in a liposome can reduce cytotoxicity and increase active ingredient efficacy.
Cancer medications are often extremely cytotoxic, for example, meaning it is particularly important to reduce cytotoxicity in cancer medication.9
Cytarabine, for example, is an anti-tumor drug with low bioavailability and blood circulation time. Severe side effects have been linked to the standalone use of the drug, prompting Depocyt® to leverage DepoFoam and liposome technology to lower the required administration frequency, increasing patient compliance.9
The antibiotic Amphotericin B is used to treat fungal infections, but it is also cytotoxic. When used alone, Amphotericin B suffers from poor absorption and can also lead to kidney function deterioration.
Encapsulating Amphotericin B in a liposome improved its macrophage absorption rate. The macrophages transported the liposomes to the infection site before steadily releasing the Amphotericin B. Removing the liposomes from the plasma increased absorption and reduced side effects.
Versatility
Liposomes carry many active ingredients, including enzymes, vaccines, and drugs.10 Liposomes’ phospholipid structure allows them to carry hydrophilic and hydrophobic nanoparticles, with hydrophilic components incorporated with the hydrophobic tails and hydrophobic components held in the liposome’s center.5,11
Liposomes are customizable nanocarriers, including in size. For example, researchers can adapt liposomes’ size using sonication, extrusion, and homogenization methods. Larger particles require larger liposomes, but smaller liposomes can better evade the immune system.8
Nanomedicine performance can also be adjusted via adjusted lipid composition, nanoparticle liposome hybrids, and adding surface modifications.3,11 For example, cholesterol is an important component of phospholipid bilayers and can affect permeability.5
Formulation parameters such as cholesterol content can be adjusted to alter a liposome’s properties. The phosphates in the liposome can carry a negative, positive, or neutral charge, impacting cytotoxicity and drug delivery.2
It is also possible to customize liposomes to alter the active ingredient’s release rate, such as adding additional lipid bilayers inside the liposome to slow this down.12
Stealth liposome technology involves the addition of polymer attachments to a liposome, better protecting the liposome from the immune system. Polyethylene glycol (PEG) is the most used polymer, with peglaytion frequently used to extend an active ingredient’s circulation time by reducing the likelihood of liposomes fusing. This technique helps to improve the delivery of the active ingredient.2,9,11
The liposome nanomedicine Doxil® contains doxorubicin. PEG is attached to the liposomes in this nanomedicine, improving its anti-tumor effect while ensuring it remains in the body longer. This allows the drug more time to reach its target.
PEG modifications are a valuable tool, but they do have several limitations. For example, patients receiving high doses of medicines like Doxil® can experience skin symptoms not associated with non-PEG liposome nanomedicines.2
Specificity
A key benefit of liposome nanocarriers is their capacity to allow the selection of disease targets. Targeting liposomes is also useful in imaging applications like ultrasounds and MRIs.11
The enhanced permeability and retention (EPR) effect is a popular passive targeting method for cancer nanomedicines. Tumors exhibit enhanced permeability from irregular blood vessels, which cause holes in the capillaries. This results in low fluid circulation in tumors and the accumulation of macromolecules.
Macromolecules, like liposomes, will accumulate more in tumor tissue than non-tumor tissue, but numerous factors prevent the effective use of EPR in nanomedicine targeting, for example, limited understanding of tumor microenvironments.13 EPR alone is typically inadequate for accurate targeting as a result.1
The use of external stimuli represents another widely used targeting method. For example, thermosensitive liposomes can be used with radiofrequency ablation to increase temperature in the target area.9 Liposomes have also been developed to employ sound as a targeting mechanism, enabling their use in ultrasound contrast agents.8
External stimuli can also be used to control drug release time,8 with pH-sensitive and temperature-sensitive liposomes exhibiting the potential for use in phase transition to control drug delivery time.10
Certain molecules can be bound to the liposome to actively target liposome nanomedicines. These molecules, called ligands, can include proteins, sugars, or antibodies.13 A combination of EPR and actively targeted liposomes can improve drug effectiveness.
Proven
The FDA and the European Medicines Agency (EMA) have approved liposomal medications for decades.2,14 The FDA approved AmBisome for treating fungal infections in 1990, for example.2
Liposome medications have also been approved for many applications, including cancer therapy drugs, vaccines, pain management, anesthesia, and treating age-related macular degeneration.2,14
Doxorubicin liposomes are one highly studied example. Despite its widespread use in cancer treatments, doxorubicin is highly cytotoxic when used alone. Encapsulating it in a liposome reduces toxicity, enabling the safer administration of higher drug doses.
Doxil®—the doxorubicin liposome nanomedicine—was approved by the FDA in 1995 and by the EMA as Caelyx® in 1996 before being listed in China in 2003.2
Liposomes have been widely proven beneficial and safe for various pharmaceuticals, but their safety continues to be re-evaluated as researchers explore new possibilities.
This is especially important as lipid nanoparticles begin to incorporate new components. Liposome nanomedicines can also benefit from the critical quality attributes already standardized for approval,1 for example, the Moderna mRNA vaccine, which was approved by the FDA in 2021.2
Future
The administration method of lipid nanoparticles presents a challenge for future researchers. Most current liposome drugs approved by the FDA utilize spinal or intravenous administration, but Arikayce®—a drug inhaled to treat the lung disease Mycobacterium avium complex—demonstrates the potential of alternative administration methods.15
Ongoing challenges in lipid nanoparticle medicine also represent opportunities for researchers to continue to explore and improve. One notable challenge lies in the effective targeting of nanomedicines, whereby current liposome nanomedicines typically depend on the EPR effect for active targeting.
Researchers could explore methods such as ligand attachments and external stimuli to better target nanomedicines.1 A nanomedicine must be close to the target area to target this, a notable challenge within current scholarship successfully.
If the nanomedicine circulates through the blood too fast, the ligand will not reach its destination, rendering the targeting mechanism useless.13 Additional research is required to better extend blood circulation and develop targeting techniques to enhance effectiveness and reduce cytotoxicity.
Lipid nanoparticles could also be adapted to accommodate individual patients as part of a personalized medicinal approach. Personalized medicine acknowledges each patient’s unique factors and aims to account for these during treatment.
The capacity of nanomedicines to better target specific conditions or areas could allow patients to receive higher dosages in line with their specific condition and open new doors in the diagnostic space.
Personalized medicine also prompts consideration of the precise tumor microenvironment when treating cancer, addressing a major issue in targeting nanomedicines. For example, a specific receptor could be attached to a liposome to target an area where the disease presents in a patient.16
Combining individual diagnostics and treatment also offers a further opportunity to customize liposomes. The same liposome could be employed in imaging and drug delivery, with this dual liposome pharmaceutical helping clinicians to know when to end a particular treatment.1
Lipid nanoparticles also have the potential to carry other types of drugs, including active proteins and gene drugs, helping to reduce the risk of denaturation and ease delivery to the cell.2 Researchers face increased challenges in this instance, as the nanoparticles could be more cytotoxic than a liposome.8
The future holds considerable promise in personalizing, improving, and adapting liposomal nanomedicines to address these challenges. The right combination of bioavailability, versatility, specificity, and proven success will be key to realizing this future.
References and Further Reading
- Giordani, S., Marassi, V., Zattoni, A., Roda, B., & Reschiglian, P. (2023). Liposomes characterization for market approval as pharmaceutical products: Analytical methods, guidelines and standardized protocols. Journal of Pharmaceutical and Biomedical Analysis, 236, 115751. https://doi.org/10.1016/j.jpba.2023.115751
- He, Y., Zhang, W., Xiao, Q., Fan, L., Huang, D., Chen, W., & He, W. (2022). Liposomes and liposome-like nanoparticles: From anti-fungal infection to the COVID-19 pandemic treatment. Asian Journal of Pharmaceutical Sciences, 17(6), 817–837. https://doi.org/10.1016/j.ajps.2022.11.002
- Akbarzadeh, A., Rezaei-Sadabady, R., Davaran, S., Joo, S. W., Zarghami, N., Hanifehpour, Y., Samiei, M., Kouhi, M., & Nejati-Koshki, K. (2013). Liposome: classification, preparation, and applications. Nanoscale Research Letters, 8(1). https://doi.org/10.1186/1556-276x-8-102
- Nasr, G., Greige‐Gerges, H., Elaı̈Ssari, A., & Khreich, N. (2020). Liposomal membrane permeability assessment by fluorescence techniques: Main permeabilizing agents, applications and challenges. International Journal of Pharmaceutics, 580, 119198. https://doi.org/10.1016/j.ijpharm.2020.119198
- News-Medical. (2019, January 30). What is a Liposome? https://www.news-medical.net/life-sciences/What-is-a-Liposome.aspx
- Deamer, D. W. (2010). From “Banghasomes” to liposomes: A memoir of Alec Bangham, 1921–2010. The FASEB Journal, 24(5), 1308–1310. https://doi.org/10.1096/fj.10-0503
- Sharma, P., Mehta, M., Tandel, N., & Tyagi, R. K. (2023). Introductory chapter: Liposome - a Versatile tool for drug delivery in nanobiomedicine. In IntechOpen eBooks. https://doi.org/10.5772/intechopen.109426
- Tenchov, R., Bird, R. E., Curtze, A., & Zhou, Q. (2021). Lipid Nanoparticles─From liposomes to mRNA Vaccine delivery, a landscape of research diversity and advancement. ACS Nano, 15(11), 16982–17015. https://doi.org/10.1021/acsnano.1c04996
- Bulbake, U., Doppalapudi, S., Kommineni, N., & Khan, W. (2017). Liposomal formulations in clinical use: An updated review. Pharmaceutics, 9(4), 12. https://doi.org/10.3390/pharmaceutics9020012
- Pande, S. (2023). Liposomes for drug delivery: review of vesicular composition, factors affecting drug release and drug loading in liposomes. Artificial Cells Nanomedicine and Biotechnology, 51(1), 428–440. https://doi.org/10.1080/21691401.2023.2247036
- Xing, H., Hwang, K. S., & Lu, Y. (2016). Recent developments of liposomes as nanocarriers for theranostic applications. Theranostics, 6(9), 1336–1352. https://doi.org/10.7150/thno.15464
- Yamauchi, M., Tsutsumi, K., Abe, M., Uosaki, Y., Nakakura, M., & Aoki, N. (2007). Release of Drugs from Liposomes Varies with Particle Size. Biological & Pharmaceutical Bulletin, 30(5), 963–966. https://doi.org/10.1248/bpb.30.963
- Bertrand, N., Wu, J., Xu, X., Kamaly, N., & Farokhzad, O. C. (2014). Cancer nanotechnology: The impact of passive and active targeting in the era of modern cancer biology. Advanced Drug Delivery Reviews, 66, 2–25. https://doi.org/10.1016/j.addr.2013.11.009
- Giordani, S., Marassi, V., Zattoni, A., Roda, B., & Reschiglian, P. (2023a). Liposomes characterization for market approval as pharmaceutical products: Analytical methods, guidelines and standardized protocols. Journal of Pharmaceutical and Biomedical Analysis, 236, 115751. https://doi.org/10.1016/j.jpba.2023.115751
- Giordani, S., Marassi, V., Zattoni, A., Roda, B., & Reschiglian, P. (2023a). Liposomes characterization for market approval as pharmaceutical products: Analytical methods, guidelines and standardized protocols. Journal of Pharmaceutical and Biomedical Analysis, 236, 115751. https://doi.org/10.1016/j.jpba.2023.115751
- Alghamdi, M., Fallica, A. N., Virzì, N. F., Kesharwani, P., Pittalà, V., & Greish, K. (2022). The promise of nanotechnology in personalized medicine. Journal of Personalized Medicine, 12(5), 673. https://doi.org/10.3390/jpm12050673
Acknowledgments
Produced from materials originally authored by Kristi Hetchler from Genizer LLC.
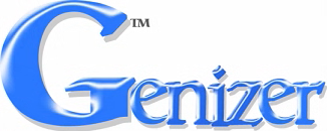
This information has been sourced, reviewed, and adapted from materials provided by Genizer LLC.
For more information on this source, please visit Genizer LLC.