Biological samples are often analyzed using atomic force microscopes (AFM), as they deliver a high resolution and are capable of operating under a range of physiological conditions. Using AFM fixed and living cells have been imaged to a large extent, leading to a better understanding of both cells and the cellular environment.
New and innovative advancements have been made in live cell imaging techniques, which have resulted in the integration of optical microscopy and AFM methods like phase contrast microscopy, DIC and fluorescence methods (TIRF, epi-fluorescence, FRET, FLIM, FCS and more), highlighting the benefits of using AFM for molecular and cell biology studies.
When compared to images acquired by traditional techniques, such as electron microscopy and optical microscopy, AFM-acquired images are completely different. In the AFM approach, a small sensor scans the cell surface, meaning both the mechanical properties and the physical shape of the cell play a role in timage formation.
This article demonstrates the standard contrast mechanisms observed in cell images acquired using AFM. It also sheds light on the differences between living and fixed cell images, including the differences between AFM and optical images.
Complementary Information about Cells and Biological Samples
Typically, most of the cell body is included in optical images of cells which is due to the nanometer scale depth of focus. While confocal optical microscopy makes it easy to acquire z-slices of the cell, tracking the exact surface of a cell can still be difficult. Contrast in such images is either the result of slight variations in refractive indices or from labeling or staining the sample.
AFM-acquired cells images reveal both surface and mechanical data. The balance between these two factors not only relies on the nature of cell sample, but also depends on the type of experiments carried out and the imaging settings used. It has been shown that AFM is ideal for analyzing the cytoskeleton and membrane surface. Imaging can be carried out in cell cultures or a buffer medium, and the images obtained provide accurate 3D data. All of this is done with no need for tedious fixing, staining or labeling processes.
The AFM imaging technique can be applied to study exocytosis or endocytosis. It can also be used to study cell-to-cell or cell-to-surface interactions. With the help of AFM instrumentation; force generation by cell movement, adhesion measurements, surface structures and cytoskeleton reorganization have all been studied in detail. In order to replicate cells using certain interactions the tip of the AFM can be easily altered. The AFM technique can be used for cell stimulation or manipulation purposes.
Optical microscopy combined with an AFM technique gives way to immense possibilities for cell microscopy. When AFM is coupled to the Zeiss Axiovert 200 inverted optical microscope with the JPK NanoWizard® instrument, users can obtain a host of complementary data. With this approach, it becomes easy to select cells and their reactions, and their status can be optically tracked.
As a result AFM can be used to study certain interactions or surface topography. Mechanical stimulation of individual cells can be done with the AFM tip, and fluorescence can be used for an optical analysis of biochemical responses.
With the combination of fluorescence labeling and AFM, the topographic features on the cell surface can be associated with labeled functional cell structures. Fluorescence techniques such as TIRF, or optical reflection techniques such as IRM, can be used for studying adhesion foci, along with quantitative force measurement by employing the AFM cantilever. For instance, the changes in cell elasticity can be studied during cell division.
The combined use of optical microscopy and AFM provides valuable data that can be integrated to obtain a better insight into cells and their environment. This integrated approach paves the way for novel experiments, which can be applied to understand more aboutcells and their reactions.
Fibroblasts
In this analysis, a fibroblast cell line was considered for introducing cell imaging with the AFM technique. These fibroblasts are characterized by a well-developed cytoskeleton that reveals a link between AFM images and images acquired through standard imaging methods. Figure 1 shows optical images of mouse dermal fibroblasts.
.jpg)
Figure 1. Optical images of fibroblasts. Upper image –brightfield/DIC. Lower image – fluorescence using FITC-labelledphalloidin, 63x oil immersion lens.
In the top image, a set of three fibroblasts produced on a glass coverslip is shown. Here, the brightfield/DIC image displays contrast from the cell edges and nucleus. A high contrast can also be seen on debris surrounding the cells.
The bottom image shows a fluorescence image, where FITC phalloidin was used to label the cytoskeletal actin; a 63x oil immersion lens was also employed. Labeling was also done to the thick stress fibers passing across the cell. For comparison, the area enclosed by the fluorescence image is highlighted with a box in the upper optical image.
The Zeiss Axiovert 200 inverted optical microscope equipped with a NanoWizard® AFM was used to acquire the images (Figure 1), and AFM can be used for scanning the cells on the coverslips, which were used for both growth and optical imaging.
AFM Imaging of Living Cells
In the case of living cells, the cytoskeleton affects the details of the AFM images. When compared to the cytoskeletal fibers, the cytoskeleton is very soft and this difference in rigidity gives a clear and visible contrast. This holds true in the case of fibroblasts that have a well-developed cytoskeleton.
.jpg)
.jpg)
Figure 2. Images of living fibroblasts using JPK NanoWizard® (upper image – surface topography, lower image – deflection signal, 80µm x 80µm scans). The deflection signal is similar to the first derivative of the topography signal.
Figure 2 shows the standard features of the AFM images of living fibroblasts. In the upper image, the nucleus dominates the topography image data and the height range across the entire cells is shown to be a few microns. The 3D topography image reveals the cells’ overall height. It is possible to view both the position and edges of the cell bodies. The cell volume, predominantly for separated cells, can be determined using the height data.
In the bottom image of Figure 2, the intricate details of the cell structure can be seen in the deflection signal images.These, in turn, correspond with the gradient of the height image and as a result appear shadowed. However, even the sharpest and tiniest features can be clearly observed in these images.
Also, the thick stress bundles of actin filaments around the edges of the living fibroblasts can be viewed more clearly. Likewise, identical features can be observed in the AFM images, similar to the labeled fluorescence image shown in Figure 1. This shows that the rigid filaments are mostly actin-based. The AFM image also reveals the details of cell boundaries as well as some rounded features relating to organelles under the surface.
Figure 3 shows a couple of smaller scans of a living endothelial cell, further underlining the benefits of the AFM resolution. Since this cell is not part of a confluent layer, it is easy to observe the texture of the petri dish in the image background.
.jpg)
Figure 3. AFM images of a living endothelial cell in plastic petri dish, taken using the JPK NanoWizard® (left image – topography, right image – deflection signal, both 28µm x 31µm).
The cell’s edge extends from the base of the image and the features of cytoskeletal filaments and parts of the cytoskeleton can be clearly observed. In contrast to optical methods, the details of the cell edge are much clearer and the structural movement of the edge of the living cell can also be examined easily. It is also possible to analyze the forces from mechanical properties of the cell, such as its adhesion and cell movement.
AFM Imaging of Fixed Cells
Cell fixation tends to make the surface of the cell membrane rigid through the crosslinking of membrane proteins. As soon as the cells are fixed, they remain in a frozen state. This makes it possible to image the cells without affecting the functions or state of the cells. The phenomenon of cell surface stiffening indicates that the underlying cytoskeleton does not significantly dominate the AFM images of fixed cells, revealing most of the details of the membrane surface like microvilli.
Figure 4 shows a couple of AFM images of a fixed fibroblast. Here, the variations between living and fixed cells can be clearly observed.
.jpg)
.jpg)
Figure 4. AFM images of fixed fibroblasts, taken using the JPK NanoWizard® (upper image – topography, lower image –deflection signal, 60µm 60µm scans).
It can also be seen that the images are not dominated by the actin filaments in the same way as in fixed cells, and more surface texture is visible from the membrane. The cells are enclosed by sharp protrusions, which are relative to microvilli on the surface of the cell.
In the top image of Figure 4, it can be seen that the nucleus height dominates the topography image, while the microvilli and cell boundaries can also be seen. Once again, the image of the deflection signal underlines the sharper and smaller features on the surface of the cell. Here, these smaller details are formed by the microvilli instead of the cytoskeleton. These images also reveal the underlying filament network, but only as a background to the other features.
Conclusion
This article has provided a better understanding of the standard features of AFM cell imaging technique, opening up new possibilities to apply this approach to other fields of experiments. AFM combined with optical microscopy extends the scope of data that can be obtained regarding the structure and function of cells.
The nature of cells used governs the complete details of cell images, and thus, the data that can be obtained from these images. While fixed and living fibroblast cell line has been highlighted here, cells with more complicated structures present a more dynamic and interesting opportunity to interpret cells and their environment.
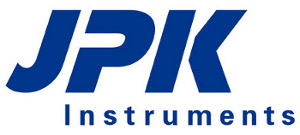
This information has been sourced, reviewed and adapted from materials provided by JPK Instruments AG - Scanning Probe Technologies.
For more information on this source, please visit JPK Instruments AG - Scanning Probe Technologies.