Atomic force microscopy (AFM) represents a better choice compared to light-based microscopy methods for the stufy of microtubules. For instance, the AFM can be used in buffers to image samples at a relatively better resolution and under different physiological conditions when compared to electron microscopy (EM).
It is a well-known fact that a large number of biological samples are heterogenic in nature and that labels are required to differentiate certain components in a system. However, such labeling methods are not available for AFM making it difficult to define structures in complicated biological systems.
This issue can be overcome using an imaging setup that integrates different types of imaging methods. For instance, an inverted light microscope can be mounted with the JPK NanoWizard® AFM, which helps in associating the images created on a sample through a range of microscopy methods.
This set up can be used for structural characterization of cell surface and also for studying each cellular components employed in in vitro assays.
This article demonstrates how the NanoWizard® AFM allows the acquisition of high quality AFM and fluorescence images of microtubules polymerized in vitro. As a rule, fluorescent imaging is used in vitro analyses of microtubule dynamics. However, the AFM technique facilitates a greater level of characterization of the microtubule structure, widening the possibilities of these in vitro analyses.
Microtubules
A cytoplasmic pool of a/b tubulin dimmers contribute to the formation of microtubules in cells. On a structural basis, microtubules constitute linear polymers of the globular tubulin heterodimers called protofilaments (Figure 1).
.jpg)
Figure 1. Microtubule structure. Microtubules have a filamentous structure, 25nm in diameter. Protofilaments, consisting of alpha/beta tubulin heterodimers, laterally associate to form the microtubule. Polymerization and depolymerization can occur at both ends with both processes being faster at the plus end of the microtubule.
A microtubule is formed when protofilaments laterally link together. The latter contains alpha/beta tubulin heterodimers. Processes like polymerization and depolymerization can take place at both ends of the microtubule, with both these processes occurring at a faster rate at the plus end of the microtubule.
Since microtubules form part of the cellular cytoskeleton, they play a major role in cellular structure. They also play a role in the guided motion of organelles, vesicles and chromosomes. this motion depends on microtubule polarity. A number of studies have been undertaken that observed in situ microtubule polymerization; however, microtubules can be polymerized and examined in vitro. This approach helps in studying the interaction of the motor proteins that play a role in the guided transport of organelles along the length of microtubules.
In these analyses, tubulin was purified from a pigs brain and was then polymerized in a ratio of 3:1 in the presence of TRITC labelled tubulin. In order to prevent depolymerization, a slowly hydrolysable GTP (GMPCPP) was used to stabilize the microtubules. The stablised microtubules were subsequently transferred to a silanized glass coverslip, and then imaged in the buffer, which is often used in microtubule assays (BRB80: 80mM PIPES pH 6.8, 1mM MgCl2, 1mM EGTA) with the aid of the JPK coverslip holder.
A 63 x 1.25 NA objective was used to collect fluorescent images, and microtubules were imaged to create AFM images. This was done by means of the intermittent contact mode with a triangular and sharpened silicon nitride cantilever, spring constant 0.32 N/m.
.jpg)
Figure 2. Fluorescent (A,B) and AFM (C) images of microtubules deposited on silanized glass, imaged in buffer BRB80. (B) and (C) both show the region highlighted in (A)
Figure 2A clearly shows the microtubules in the fluorescent image. Here, the brighter lengths marked in white arrows show where the microtubules have begun to bundle. A light speckled appearance can be observed in certain microtubules, which can be attributed to a combination of labeled and non-labeled tubulin. With the help of AFM, an area was chosen for imaging (highlighted as a white square box in Figure 2A) but here the microtubules did not appear to be bundled.
Figure 2B shows an electronic zoom of the related region of the light micrograph. However, in the AFM image acquired from the same sample regions, more of the microtubules structural properties can be seen when compared to the image acquired using epi-fluorescence. The AFM image also clearly shows breaks in the microtubule that cannot be seen in the epi-fluorescent image (Figure 2B and C, white arrows). The brighter fluorescent spots also match with the large structures on the microtubules, as shown in Figure 2B and C marked in red arrows.
Imaging biological components with fluorescence microscopy does not produce structural data at less than 250 nm resolution, making it difficult to define the surface structure of biological components. Although the images demonstrate the interaction between microtubules and fluorescently labeled components, the binding effect of other compounds like salts to the surface could not be observed with light microscopy.
Microtubules are vulnerable to the salt composition of their environment as they exhibit a high affinity for salt ions. Microtubules stabilized by Taxol were allowed to stick to silanized glass and then dried. The untreated microtubule surface seems to be smooth, as shown in Figure 3A.
As soon as a salt-containing buffer is added, the microtubule surface changes. In another case, buffer is used to wash the microtubules followed by ultrapure water prior to imaging. This results in a globular appearance (Figure 3B). However, this change in the surface structure becomes more pronounced when a salt-containing buffer is used to wash microtubules followed by drying without the ultra-pure water washing step (Figure 3C).
Since the microtubule activity related to motor proteins is influenced by salt concentration, the local salt concentration at the microtubule surface can also impact the in vitro study of motor protein activity.
.jpg)
Figure 3. AFM images of taxol-stabilized microtubules in air. Microtubules were untreated (A), treated with salt buffer (B), or treated with salt buffer and then rinsed (C).
Figure 4 shows a 3D representation of Figure 3A, where striations along the length of the microtubule can be seen. These striations probably indicate the presence of protofilaments in the microtubule.
.jpg)
Figure 4. Three-dimensional representation of the microtubule image shown in Figure 3A.
Dynamic instability defines the polymerization of microtubules, where depolymerizing microtubules exist along with polymerizing microtubules. In the case of in vitro analyses, the absence or presence of different microtubule-related proteins can change the rates of depolymerization and polymerization. This shows that such proteins play a key role in the regulation of microtubule dynamics throughout the course of different stages of the cell cycle. The dynamics of microtubules as well as the directional activity of motor proteins along the microtubules can be effectively examined in vitro.
Light microscopy is often applied for studying the movement and interaction of different motor proteins along the length of microtubules in addition to the interaction of microtubule destabilizers and stabilizers. Such experiments, however, can give way to variable outcomes, especially in the case of proteins that stick to the microtubule ends. Protein binding along the microtubule length can be seen in analogous samples, in addition to protein binding at the end of microtubules. Samples when imaged with AFM clearly show breaks in the microtubules.
The salt composition of the buffering solution as well as its ionic strength affect the movement of specific microtubule related motor proteins. This may be due to the fact that differences in microtubule surface-related ions contribute to inaccurate results between experiments.
AFM’s high spatial resolution combined with light microscopy can be applied to study this in vitro system in a more detailed way. AFM can be used to characterize the structure of the microtubule, whilst confocal, epi-fluorescent, or total internal reflection fluorescence microscopy can be used to define the corresponding microtubule-related protein dynamics. Imaging a sample with both AFM and epi-fluorescence techniques may also allow structural characterization. AFM can possibly be used for imaging breakdown-products subjected to microtubule destabilizers, offering more data regarding the action mode of destabilizing proteins.
Conclusion
Light microscopic techniques are traditionally used for studying microtubules in vitro, but such methods have significantly limited the scope of such analyses. Such experiments provide data regarding the function and dynamics of microtubules, but they fail to offer high resolution structural data. An inverted light microscope equipped with the NanoWizard® AFM opens up many possibilities for in vitro analyses, with no optical limitations.
Several methods such as IRM, CLSM, TIRF, FRET, including the use of phase contrast or DIC condenser methods can be simultaneously employed with the AFM technique. All of these factors make NanoWizard® AFM a true BIO-AFM imaging instrument.
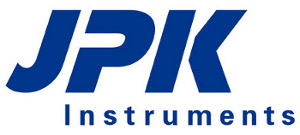
This information has been sourced, reviewed and adapted from materials provided by JPK Instruments AG - Scanning Probe Technologies.
For more information on this source, please visit JPK Instruments AG - Scanning Probe Technologies.