Advanced three-dimensional electron microscopy (3DEM) techniques for scanning electron microscopes (SEM) have been developed over the past several years. These novel tools have the ability to perform imaging at spatial resolutions better than that of optical microscopy by over an order of magnitude.
A key 3DEM technique known as serial block-face scanning electron microscopy (SBFSEM) has continued to widen the 3D resolution limits by obtaining serial images from volumes, i.e. slices, thinner than 15 nm. Here, the imaging involves detecting the backscattered electrons using a low kV optimized detector. Specific characteristics are mandatory to enhance the spatial resolution in all three axes: the principal criterion is matching the accelerating voltage to the slice thickness. SBFSEM samples consist of plastic embedded biological samples that are stained with heavy metals such as uranium and lead.
A major prospective limitation in obtaining a SBFSEM image is sample charging. This can be compensated by carrying out imaging with nitrogen gas or water at low pressure. In addition, if the imaging is carried out at low accelerating voltage, the need for compensation and charging effects are significantly minimized or eliminated.
Experimental Method
For the purpose of determining the penetration depth of the electron beam at two disparate beam energies, two Monte Carlo simulations were carried out. A blank epoxy section was overlaid on top of a block-face specimen to confirm the Monte Carlo simulations with the experimental data.
Figure 1A illustrates an image obtained at an accelerating voltage of 3.0 kV. The left side of the image includes a 50 nm thick section overlaid on the right side of the image. At the beam energy of 3.0 kV, the electrons possess adequate energy for penetrating the blank resin section and generating a signal from the block-face below.
Figure 1B illustrates the same experimental layout showing images obtained with the beam energy of 2.0 kV at which the electrons do not possess adequate energy for penetrating the 50 nm blank resin section. Thus, no signal is generated from the block-face below. Both of the attached Monte Carlo simulations confirm the electron penetration that is indicated by the backscattered electron trajectories marked in red.
.jpg)
Figure 1. Monte Carlo simulations with corresponding SBFSEM images, demonstrating signal depth through a 50 nm slice of blank resin on top of a block-face.
It is important to understand the penetration depth while collecting serial images that are thinner than 50 nm because much lower accelerating voltages are needed to maintain a Z resolution that is better than the slice thickness.
As shown in Figure 2, two additional Monte Carlo simulations carried out for cutting 15 and 5 nm images imply that the corresponding beam energies are 1.0 and 0.5 kV, respectively.
.jpg)
Figure 2. Monte Carlo simulations demonstrating the depth of backscattered electron signal at 1.0 and 0.5 kV. The signal depth at 1.0 kV does not exceed 15 nm, while the signal depth does not exceed 5nm at 0.5 kV
As illustrated in Figure 3, when the imaging is carried out at low accelerating voltages, a principal advantage is that charging becomes a smaller potential problem and is completely eliminated if the beam energy is equal to or lesser than 1.5 kV. When charging is reduced, image acquisition in high vacuum is enabled, thus enhancing the signal-to-noise ratio and hence, generating images of higher quality at a shorter time period.
By using suitable staining, even dense biological samples such as liver or brain tissues can be easily imaged in high vacuum even at higher voltages of 2.0 kV. Samples such as plant or cell monolayers that contain large fields of open unstained resin more easily undergo charging and therefore, can be imaged at low accelerating voltages. Figure 3 depicts an example in which imaging is carried out at 1.4 kV in high vacuum with minimal charging.
.jpg)
Figure 3. Arabidopsis SBFSEM images acquired with a 3View system on a Zeiss Merlin in high vacuum at 1.4kV. Image A was acquired with 50nm pixels, image B with 10nm pixels, and image C with 5nm pixels.
Figure 4 illustrates the image quality acquired by using the Gatan backscattered electron detector at 1.0 and 0.9 kV.
.jpg)
Figure 4. Mouse kidney SBFSEM images acquired with a 3View system on an SEM in high vacuum. Image A is an extract of a 4k x 4k image acquired at 900 V. Image B is an 8k x 8k image acquired with 10nm pixels at 1.0 kV.
The Gatan detector is optimized for carrying out imaging at low accelerating voltages and can produce images with high signal-to-noise ratio even if the imaging is carried out at a beam energy equal to or lesser than 1.0 kV.
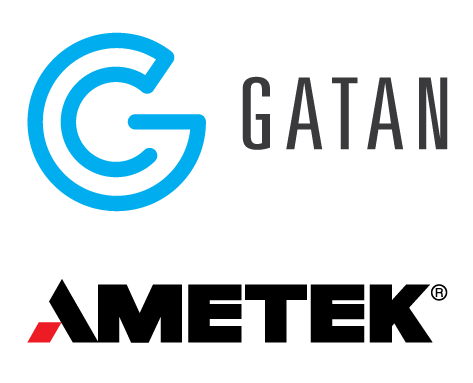
This information has been sourced, reviewed and adapted from materials provided by Gatan, Inc.
For more information on this source, please visit Gatan, Inc.