Magnetic microspheres are composite structures created by integrating magnetic inorganic particles with organic polymers.
These magnetic composite microspheres not only retain the characteristics of standard polymeric microspheres but also demonstrate magnetic responsiveness. They can be modified with functional groups (such as -OH, -COOH, -CHO, -NH2, etc.) through methods like copolymerization and surface modification. Furthermore, they demonstrate directional functionality when subjected to an external magnetic field.
Magnetic microspheres have been widely used in several sectors, such as biomedicine, cell biology, and separation engineering, since the 1970s. To achieve effective magnetic separation, ideal magnetic microspheres must have a substantial specific surface area, consistent surface ligand connections, and a steady separation environment.
In the study outlined in this article, the BeNano 90 Zeta was utilized to track the zeta potential and size changes of magnetic microspheres at various pHs in response to temperature changes.
Zeta potential serves as a crucial indicator of particle system stability. A higher absolute value of zeta potential signifies stronger repulsive forces between particles, thereby indicating greater system stability.
Instrumentation
The BeNano 90 Zeta was used to evaluate the size, molecular weight, and zeta potential using dynamic light scattering (DLS), static light scattering (SLS), and electrophoretic light scattering (ELS) technologies.
The sample was illuminated with a 50-mW solid-state laser beam at 671 nm. An avalanche photodiode (APD) detector and fiber were then utilized to capture scattered light signals at 12° for zeta potential measurement and 90° for size and molecular weight measurements.
Theory
Dynamic Light Scattering (DLS)
DLS is a technique that measures the diffusion behavior of particles dispersed in liquids. The continuous random movement of suspended nanoparticles in a liquid medium is referred to as Brownian movements, and the speed of these motions is correlated with the sizes of the particles. The Brownian motion of the particles causes the scattered light intensity to vary when a laser beam illuminates the nanoparticles in suspensions.
After that, APD detects the intensity of the spread light and uses the correlator to convert it to a correlation function. The diffusion coefficient (D), which characterizes the speed of Brownian motion, is therefore determined by examining the correlation function. The Stokes-Einstein equation is used to get the hydrodynamic diameter (DH):
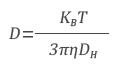
where kB is the Boltzmann’s constant, T is the temperature, and η is the viscosity of the solvent.
Electrophoretic Light Scattering (ELS)
During an ELS experiment, the sample is exposed to a laser beam, and the scattered light is monitored at a forward angle of 12°. The sample solution or suspension is exposed to an electric field at both ends of the sample cell, which causes the electrophoretic movement of the charged particles. The Doppler effect causes a frequency shift in the scattered light as a result when compared to the incident light.
PALS analysis converts dispersed light signals with frequency shifts to phase shifts. The electrophoretic mobility µ, or velocity of electrophoretic movement per unit electric field, is determined by the phase plot. Henry's equation establishes a relationship between the electrophoretic mobility (µ) and its zeta potential (ξ):
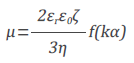
where k is the reciprocal Debye length, α is the particle radius, ε0 is the solvent dielectric constant in vacuum, εr is the relative dielectric constant, η is the solvent viscosity, and f(kα) is the Henry function. The ratio between the particle radius and the double layer thickness is denoted by kα.
Zeta potential is a vital indicator of particle system stability. A larger zeta potential suggests stronger repulsive interactions between particles, leading to increased system stability.
Experiment
The measured magnetic microsphere sample was commercially sourced and underwent surface modification through copolymerization or chemical bonding, resulting in undisclosed functional groups.
After being diluted with distilled water, the pH of the magnetic microsphere stock solution was found to be 6. A certain quantity of magnetic microspheres was diluted to a pH of 3 using an HCl solution and then to a pH of 9 using a NaOH solution.
Separate samples with pH = 3, 6, and 9 were added to PS cuvettes. The temperature was raised from a starting point of 25 °C to a final temperature of 60 °C, with a 1 °C temperature interval, to measure the sizes.
Furthermore, by utilizing a temperature interval of 1 °C, an initial temperature of 25 °C, and a final temperature of 0 °C, size measurements with decreasing temperature were carried out.
To assess the trend of zeta potential vs temperature, samples with pH values of 3, 6, and 9 were introduced into folded capillary cells. The size vs. temperature trend measurement and the zeta potential measurement use the same temperature parameters.
Results and Discussion
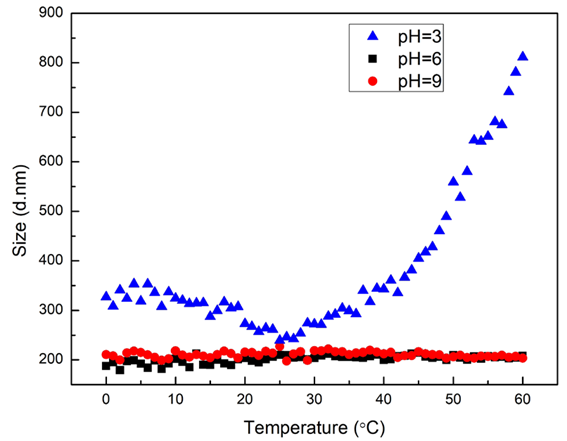
Figure 1. Size vs. temperature trend results of magnetic microspheres with pH = 3, 6, and 9. Image Credit: Bettersize Instruments Ltd.
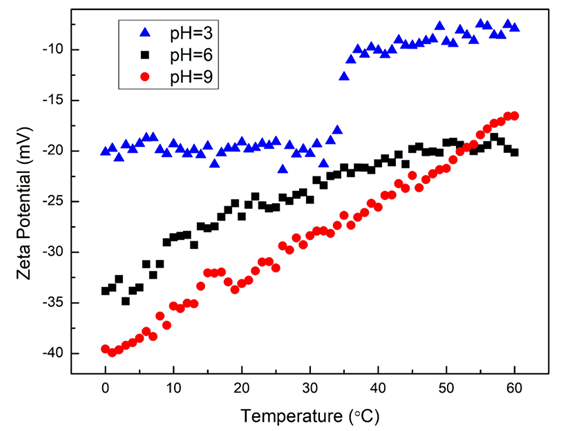
Figure 2. Zeta potential vs. temperature trend results of magnetic microspheres with pH = 3, 6, and 9. Image Credit: Bettersize Instruments Ltd.
The temperature-dependent zeta potential and size differences of magnetic microspheres at various pH values (3, 6, and 9) are displayed in Figures 1 and 2. The Z-average size of all three samples is around 200 nm at 25 °C, and the negative zeta potential indicates negatively charged particles. At 25 °C, the absolute value of the zeta potential increases with increasing pH.
The size of magnetic microspheres in settings with pH values of 6 and 9 is generally steady within the range of 0-60 °C. The sensitivity of particle size to temperature is stronger at pH 3, with the lowest particle size occurring at around 25 °C. Particle size increases are induced by both heating and cooling, suggesting the formation of aggregates in the system.
The absolute zeta potentials at pH 6 and pH 9 are higher than those at pH 3 at the same temperature across the temperature range of 0 to 60 °C. Stronger interactions between the particles are indicated by a higher zeta potential, which increases the stability of the particle system and lowers the possibility that large particle aggregates could develop as a result of external stimuli.
![Size and zeta potential results of magnetic microspheres at different temperatures with]()
Figure 3. Size and zeta potential results of magnetic microspheres at different temperatures with pH=3. Image Credit: Bettersize Instruments Ltd.
Figure 3 illustrates the temperature-dependent variations in size and zeta potential of magnetic microspheres at pH 3. It is noticeable that at 25 °C, the Z-average size tends to increase with both heating and cooling processes, particularly during the heating phase. Beyond 34 °C, there is a sudden change in zeta potential, decreasing sharply from -17.99 mV to approximately -12.7 mV.
Simultaneously, there is a rapid increase in particle size observed. This phenomenon further emphasizes the importance of evaluating system stability through zeta potential, where a decrease indicates an unstable state and a higher likelihood of particle aggregation.
Conclusion
The BeNano 90 Zeta was used to assess the zeta potential and size of a sample of magnetic microspheres. The study looked at several temperature conditions and pH levels (3, 6, and 9). The results indicate that the magnetic microsphere system’s stability is particularly strong at pH 6 and 9, with minimal variation in particle size as temperature changes. However, the stability of the system is more vulnerable to changes in temperature at pH 3.
The observations suggest that under varying pH and temperature conditions, particle systems with zeta potential absolute values exceeding 20 mV exhibit enhanced stability.
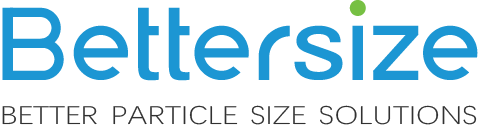
This information has been sourced, reviewed and adapted from materials provided by Bettersize Instruments Ltd.
For more information on this source, please visit Bettersize Instruments Ltd.