Silica thin films templated by two triblock copolymers (P123 and F127) from the pluronics family and having the p6m two dimensional symmetry were investigated by X-ray Reflectivity (XR) before and after removing the surfactant from the silica matrix. The analysis of XR curves by the matrix technique provides information about the average electron density of the films, the wall thickness, the electron density of the walls, the radius of the pores and subsequently the porosity of such mesoporous films. Differences arising from templating the silica matrix with these two surfactants are reported and discussed.
Introduction
The synthesis of surfactant-templated silicate materials has developed rapidly over the past decade. [1] The uniform controlled pore sizes created in the amorphous silicate framework by this method show promise as catalyst supports, sensors, filtration membranes and in a variety of optoelectronic applications. Formation of these materials as thin films has become an active area of research for which X-ray reflectivity can be used to reveal structural information.[2, 3] Among the surfactants, nonionic triblock copolymers of the pluronic family are widely used as templating agents mainly because they enable structures with larger pores and walls than the those obtained when CTAB cationic surfactants are used to direct the structure. [5] Highly organized thin films with two dimensional (2D) hexagonal or cubic arrays of pores were made successfully with block copolymer templates and have been characterized by TEM, Grazing Incidence Small Angle Scattering (GISAXS) and x-ray scattering.[6]
Recently we have shown that the X-ray Reflectivity (XR) and GISAXS is a powerful combination to study the structure of these materials.[7] By modeling the electron density profile of such films, the size of the pores, the wall thickness but also the porosity and the surface area could be evaluated.
In this work, we use the same approach to compare the effect of the two templating agents, namely the P123 and F127 of the pluronic family, on the structure of thin silica films. The two surfactants are made of the same hydrophobic polypropylene oxide (PPO) core attached on both sides with polyethylene oxide (PEO) hydrophilic arms. They only differ by the length of the hydrophilic arms that are composed of 20 (EO) monomers for P123 and 106 (EO) monomers for F127 while the core is made of 70 (PO) monomers. One may infer the structural organization of such films will depend on how the hydrophilic arms may interact with the silica matrix. Since F127 possesses longer hydrophilic arms than P123 one could expect that templating the silica with these two surfactants will cast some light on the role of the long hydrophilic arms on the structural organization of such films.
Methods and Materials
Films templated by P123 were made from initial sols prepared in two steps as described recently [8]. Similarly, films templated by F127 were made in two steps according to the following procedure.
First, 4g of tetraethoxysilane (TEOS) and 1.76g of HCl 0.055M were stirred for 30min. at room temperature. 18g of ethanol and 1.14g of F127 were then added to this mixture and the solution was stirred at room temperature for 30min to 1h. From the resulting sol, thin films were dip-coated at a constant withdrawal velocity of 14cm/min on clean glass or silicon substrates. The final sols had molar composition 1 TEOS : 72 C2H5OH : 21 H2O : 0.022 HCl : 0.012 P123 and 1TEOS:EtOH:5H2O:F127. The composition of the sols was adjusted to make films about 100nm thick.
For both surfactants two identical films were prepared. One of the films was rinsed thoroughly in ethanol for 3h to remove the surfactant so as to produce a mesoporous film and the other was not processed. ƒ
During the dip-coating the relative humidity RH was fixed at 60% for P123 and 30% for F127. We found indeed that F127 templated films were not highly organized if the humidity was kept at 60% during the dip-coating. Grazing incidence measurements [8] carried out on both systems show that the films had a distorted 2D hexagonal p6m symmetry (i.e. the cmm symmetry) before and after removal of the surfactant ascertaining that the rinsing procedure was not delaminating the films. This structure is presented in Fig. 1 together with the characteristic parameters that are of interest in the following analysis.
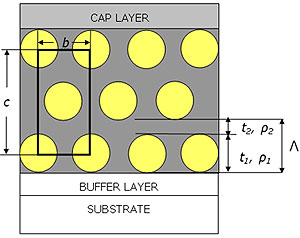
|
Figure 1. Stylised representation of silica templated thin films. Films are considered to be made of a layer 1 either composed of surfactant/pores and silica (respectively before rinsing and after rinsing) of thickness t1 electron density ρ1 and a roughness σ1 and of a silica layer 2 of thickness t2 with an electron density ρ2 and a roughness σ2. The in-plane spacing between pores or micelles is denoted b. For clarity the figure shows only 3 layers out of the N=8 layers really present in the films. The roughness of the layers is not shown in the picture. Films are supported by a glass substrate and silica cap and buffer layers are also introduced in the model.
|
XR measurements were obtained using a wavelength of 1.54Å on the reflectometers of ANU [9] and Université du Maine. Measurements on a film containing occluded surfactant were not performed in air, but in dry N2 for a rinsed film to prevent moisture ingress into the porous framework. Micro-Raman experiments were performed at room temperature using a Jobin-Yvon T64000 Raman spectrometer equipped with a confocal microscope.
|
Results
As shown in Fig. 2, the XR patterns exhibit for both films before and after rinsing typical Kiessig fringes and Bragg peaks that are characteristic of highly organized thin films. The Kiessig fringes arise from the finite thickness of the film whereas the Bragg peaks come from a periodically repeated motif inside the film. The similar overall features observed before and after rinsing confirm that the films are not delaminated during the rinsing process. Nevertheless the rinsed films appear to be shrunken compared to the as prepared one as evidenced by the shift of the Bragg peaks towards higher q wave vector transfers together with the increase of the Kiessig fringes periodicity. This effect is more pronounced in the F127 templated film.
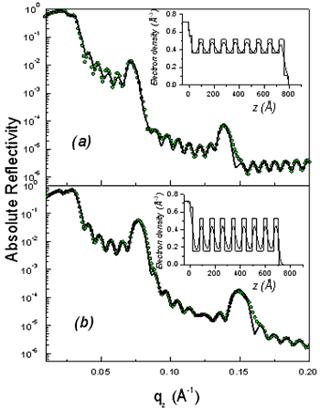 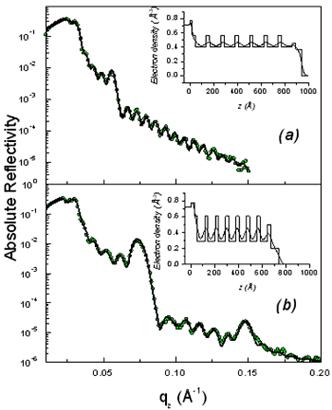
|
Figure 2. Absolute reflectivity curves of the initial (a) and rinsed films (b) for P123 (above) and F127 (above). The inset gives the electron density profile obtained from a fit via the matrix technique to the experimental data. The modifications induced by the rinsing procedure are obvious both on the electron density profiles and on the average critical wave vector.
|
From the location of the Bragg one can immediately state that before and after rinsing the electron density respectively is periodic with a period Λ1= 9.0 and 8.4nm for the P123 films and Λ2= 12.0 and 8.75nm for the F127 films. As shown in Fig. 1 the period, Λ, that is found here defines half of the unit cell, c, along the direction normal to the surface of the film. Note that the period can be either found from the distance between two consecutive peaks or from the location of the first peak provided the q position is corrected from the refraction effect ( ). Without modelling the structure, we find that before rinsing the F127 film exhibits a much larger period than the P123 one; nevertheless after rinsing the two periods become almost the same. One can also observe that the intensity of the Bragg reflections increased after rinsing the films. This behavior is expected since the removal of the surfactant from the silica matrix induces a higher electron density contrast between the silica matrix and either the surfactant or the pores. This observation proves that the rinsing procedure we have used is quite efficient to remove the surfactant. This was further confirmed by the Raman analysis shown in Fig. 3. The signal of the H-Csp3 stretching bands related to the presence of P123 and F127 (or possibly to residual Si-OC2H5 groups) inside the film decreases drastically after rinsing. From the integrated intensity of these bands, one can conclude that about 91% of the CH2 and CH3 moieties were removed.
Finally one can also observe in the XR curves exhibit two different critical qc: the first one corresponds to the average electron density of the film whereas the second one is that of the substrate (~ 0.0315 Å-1). A comparison of the two panels in each figure clearly shows that removing the surfactant has a strong effect on the average electron density of the film. For P123 and F127 the respective shift of the critical vector, qc, from 0.0243Å-1 to 0.0206Å-1 and 0.026Å-1 to 0.0232Å-1 after rinsing is significant meanwhile the substrate qc remains the same for both samples. This change can be related to the mesoporosity of the rinsed films [8].
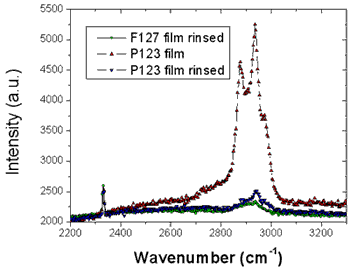
|
Figure 3. Raman scattering of the CH2 stretching bands before and after rinsing the P123 and F127 templated silica thin films showing that the surfactant has been efficiently removed. The measurements were carried out under a microscope directly on the films and the intensities were normalized for comparison by the signal of nitrogen at 2320cm-1.
|
Further quantitative information requires analyzing the experimental data via an inferred electron density profile that can be refined by a least-square fit to the data using the so-called matrix technique. The inferred model consisted in 2 stacked layers that were repeated N times as shown in Fig. 1. In this model, the thickness t1 defines both the radius of the surfactant micelle before rinsing and the pore diameter after rinsing. All the parameters were adjusted by a fit to the experimental data and are reported in Table 1 and 2. The electron density profiles were slightly different for both sets of films. In particular the two last layers were separately treated as cap layers in the F127 samples and the silica walls are put directly in contact with the silicon substrate (i.e. layer 1 becomes layer 2 and vice versa see insets of Fig.2 and 3).
The fitted density profiles (in the insets of Fig. 2) show how the electron density is modified by the removal of the surfactant while maintaining the N=8 sequence. It can be seen that in both set of films the silica walls (layer 2) have a similar electron density before and after rinsing with a slight densification after rinsing. This shows that the rinsing procedure maintains the silica walls, thus providing a mesoporous film of good mechanical properties. The wall density, 0.52 (0.53)e-/Å3 for P123 films and 0.57(0.60)e-/Å3 for F127, is however smaller than the one of bulk silica which is 0.72e-/Å3.[10] This clearly shows that the walls are not made of classical bulk silica but are either microporous or gel-like. Assuming microporous silica, we can infer the microporosity of the walls is given by , yielding a for P123 films and 20% for F127 films. This in turn yields an average mass density of the walls μwall=1580kg/m3 for P123 films and 1760 kg/m3 for F127 films (less that the one of pure silica μ=2200kg/m3).
Table 1. Parameters obtained from the fits to the experimental data of the initial and rinsed P123 films that were dip-coated on a glass substrate. Films are considered to be made of two layers that are repeated N=8 times. Cap and buffer layers of silica are also introduced in the model. For each layer we adjust the critical wave vector qc (namely the electron density ρ), the interfacial roughness σ and the thickness t. The first number is the one related to the initial film while the second is the one for the rinsed film. Parameters denoted with the * subscript were kept fixed during the fitting procedure.
|
qc (Å-1)
|
0.032
|
0.0278/0.0302
|
0.0224/0.0149
|
0.0270/0.0273
|
0.012/0.015
|
ρ(e-/Å3)
|
0.73*
|
0.56/0.65
|
0.36/0.16
|
0.52/0.53
|
0.10/0.16
|
σ (Å)
|
1.5*
|
6.5/ 8
|
11.2/10.3
|
18.1/18.8
|
3.75/4.1
|
t (Å)
|
-
|
22.9/22.4
|
55.6/52.9
|
36.1/32.4
|
33.7/10.1
|
Table 2. Parameters obtained for the F127 films
|
qc (Å-1)
|
0.0317*
|
0.0331
/0.0331
|
0.0283
/0.0292
|
0.0237
/0.200
|
0.025
/0.0265
|
0.0229
/0.017
|
ρ
(e-/Å3)
|
0.73*
|
0.78
/0.78
|
0.57
/0.60
|
0.52
/0.284
|
0.44
/0.50
|
0.37
/0.20
|
σ (Å)
|
2.5*
|
8/ 9
|
34/14.4
|
14/26
|
14/21
|
17/25
|
t (Å)
|
-
|
22.9/28.1
|
29.5/27.0
|
91.3/60.6
|
27.7/33.2
|
48.6/67.7
|
The electron density of the porous layer 1 exhibits on the contrary a drastic decrease from 0.36 to 0.14e-/Å3 for P123 and from 0.52 to 0.28 e-/Å3 for F127 after rinsing as expected form the removal of the surfactant. In addition this layer contracts by about 30% in the case of F127 films whereas it does not vary much in the P123 films.
From the parameters reported in tables 1 and 2, one can calculate the average electron density <ρ> of each film and compare it to the unbiased experimental value measured at the critical angle of external reflection as recently reported. The average electron density of the film is by definition
Eq. 1
in which re=2.8510-15m is the classical radius of the electron. Substitution of the fitted parameters into Eq. 1 gives a density 0.30 e-/Å3 (i.e. <qc>=0.0206 Å-1) for the P123 rinsed film and 0.42 e-/Å3 (i.e. <qc>=0.0243 Å-1) for the as P123 deposited film. These calculated values are in perfect agreement with the experimental values of qc shown in the bottom insets of Fig. 2. The fitting analysis for q >qc confirms the simple analysis of the average electron density obtained for q < qc. After rinsing, the pore diameter was found to be 5.3±1nm. In the case of the F127 sample, we obtain a density 0.38 e-/Å3 (i.e. <qc>=0.0232 Å-1) for the rinsed film and 0.53 e-/Å3 (i.e. <qc>=0.0273 Å-1) for the as deposited film. Once again these values are in very good agreement with what is observed in Fig. 3 although a small discrepancy is found for the as deposited film.
The fitted parameters of the rinsed films allow obtaining the mesoporosity. It relates to the parameters t1, t2, ρ1 and ρ2 by the following expression
Eq. 2
From this equation we find that Φmeso=43% for the P123 film and 36% for the F127. One can note that the porosity is also given by , a result that is fully consistent with Eq. 2 when substituting by its expression (Eq. 1).
|
1. Frye G.G.,Ricco, A.,Martin, S.G.and Brinker, J.C., "Characterization of the surface area and porosity of sol-gel films using SAW devices", Mat. Res. Soc. Symp. Proc., 121, 349-354, 1988.
2. Gibaud A., "Specular reflectivity from smooth and rough surfaces, in X Ray and Neutron Reflectivity: Principle and Applications", Eds, Daillant J.and Gibaud A., Springer, Paris, 87-115,1998.
3. Gibaud A.,Dourdain, S.and Vignaud, G., "Analysis of mesoporous thin films by x-ray reflectivity, optical reflectivity and grazing incidence angle x-ray reflectivity", Appl. Surface Science, in Press,
4. Bolze J.,Ree, M.,Youn, H.S.,Chu, S.H.and Char, K., "Synchrotron X-ray reflectivity study on the structure of templated polyorganosilicate thin films and their derived nanoporous analogues", Langmuir, 17, (21), 6683-6691, 2001.
5. Soler-Illia G.,Crepaldi, E.L.,Grosso, D.and Sanchez, C., "Block copolymer-templated mesoporous oxides", Current Opinion In Colloid & Interface Science, 8, (1), 109-126, 2003.
6. Soler-Illia G.,Crepaldi, E.L.,Grosso, D.,Durand, D.and Sanchez, C., "Structural control in self-standing mesostructured silica oriented membranes and xerogels", Chemical Communications, (20), 2298-2299, 2002.
7. Gibaud A.,Baptiste, A.,Doshi, D.A.,Brinker, C.J.,Yang, L.and Ocko, B., "Wall thickness and core radius determination in surfactant templated silica thin films using GISAXS and X-ray reflectivity", Europhysics Letters, 63, (6), 833-839, 2003.
8. Dourdain S.,Bardeau, J.F.,Colas, M.,Smarsly, B.,Mehdi, A.,Ocko, B.M.and Gibaud, A., "Determination by x-ray reflectivity and small angle x-ray scattering of the porous properties of mesoporous silica thin films", Applied Physics Letters, 86, (11), 2005.
9. Brown A.S.,Holt, S.A.,Saville, P.M.and White, J.W., "Neutron and X-ray reflectometry: Solid multilayers and crumpling films", Australian Journal Of Physics, 50, (2), 391-405, 1997.
10. A. Gibaud, in "X-ray and Neutron Reflectivity: Principles and application", edited by J. Daillant and A. Gibaud, Springer Paris (1999), p. 87-115.
|