Many areas of biological, chemical and medical research and development (R&D) use fluorescent macromolecules and nanoparticles. The basic physical characterization of such materials is regarded as highly important for R&D. However, interference of the fluorescent emission by detectors designed to measure scattered light makes it undoubtedly challenging.
Wyatt Technology has designed instrumentation which offers multiple avenues to characterization of fluorescent samples; the current article describes how Wyatt’s SEC-MALS, DLS and PALS detectors can overcome the major challenges of fluorescence. Also, Wyatt also offers several non-light scattering technologies that are able to deal with such samples; these too will be described.
Introduction
The amount of light scattered by a solution of nanometer-sized particles (e.g., polymers, proteins, or nanoparticles) is 0.01-100 ppm relative to the incident light beam. Detectors of multi-angle light scattering (MALS), dynamic light scattering (DLS), and phase analysis light scattering (PALS) must be very sensitive to accurately characterize the molar mass, size, and zeta potential of nanometer-sized entities. Although these instruments are designed to abolish interference from stray light scattered by the flow cell or cuvette walls, the sample itself emits light which is typically detected as scattered light.
Challenges of Fluorescence in Light Scattering
Fluorescence is found in many types of macromolecules and nanoparticles, including naturally occurring polymers, labeled proteins intended for research techniques, and tagged nanoparticles used for medical diagnostics, targeted cancer tissue imaging or environmental monitoring and remediation.
.jpg)
Every fluorescent molecule has a spectral range of fluorescent excitation between λexc,min and λexc,max, and a range of fluorescent emission between λemission,min and λemission,max. Depending on the position of λexc relative to the emission band, the actual emission spectrum may start at λexc or at λemiss,min. The difference between the excitation wavelength λexc and the shortest emission wavelength is referred to as the ‘Stokes shift’.
Numerous techniques for basic characterization of polymers, proteins, and nanoparticles utilize analytical light scattering. For instance, size exclusion chromatography with multi-angle static light scattering (SEC-MALS) determines distributions of molecular weight, root mean square radius, and conformation by combining separation by size-exclusion chromatography with absolute molar mass and size analysis by MALS. DLS is frequently used to determine the hydrodynamic radius of proteins and nanoparticles, and PALS determines zeta potential which is associated with charge and colloidal stability.
.jpg)
.jpg)
Figure 1. Top: Absorption takes place at higher energy (shorter wavelength) than fluorescent emission at lower energy (longer wavelength). Bottom: Illustration of a fluorescent molecules’ excitation and emission spectra.
To measure scattered light, an incident laser beam with a particular wavelength must impinge on the sample. This wavelength is referred to as λlaser. If λlaser is within the fluorescent sample’s band of excitation, light is absorbed and re-emitted as fluorescence; λexc is equal to λlaser. If the detector is overwhelmed by light arising from fluorescence, or if the signal amplitude is increased arbitrarily by fluorescence relative to the purely scattered intensity and does not fully saturate the detector, the analysis will be disrupted or tainted. Specifically, MALS analysis is tainted when the total measured intensity is skewed by detection of fluorescing photons. Meanwhile DLS avalanche photodiodes (APDs) are saturated by fluorescence and thus cannot detect individual photons, which is needed for DLS autocorrelation analysis.
Overcoming fluorescence to perform reliable characterization of samples can be achieved in numerous ways:
1.By preventing the excitation of fluorescence
2. By preventing fluorescent radiation from reaching the detector
3. By using a light-scattering technology that is not adversely impacted by fluorescence
4. By using a technique that does not rely on light scattering
Wyatt Technology employs all four methods, in various configurations, in order to enable optimal characterization of fluorescent macromolecules and nanoparticles. These methods, and their benefits and limitations, are discussed below.
1. Preventing the Excitation of Fluorescence: Wavelength Options
Fluorescence can only occur when λlaser is within the sample’s band of excitation. Thus, fluorescence can be prevented if an appropriate laser wavelength is used.
MALS:
Wyatt MALS detectors e.g. DAWN®, miniDAWN® and µDAWN®, are equipped with 660 nm wavelength lasers (give-or-take a few nanometers between individual instruments), which is well below the excitation band for most macromolecules and nanoparticles. Fluorescence is restricted to a relatively small class of naturally fluorescent polymers (e.g. lignin) and a small number of commonly used protein tags (e.g. Cyanine 5) for these instruments.
Although a shorter wavelength will fall outside the excitation band in some cases, for the most part a longer wavelength is preferable. This is because long-wavelength photons have lower energy than short-wavelength photons, and therefore have a significantly reduced probability of excitation. The DAWN 660 nm laser has the option of being replaced with a 785 nm laser. This wavelength (785 nm) is well below the excitation band of almost all fluorescent dyes used for protein labeling and fluorescence resonance energy transfer (FRET); in fact, use of a 785-nm laser prevents fluorescence entirely for the vast majority of such molecules. This means that even if a dye molecule emits a wavelength of less than 660 nm, it is very unlikely that it will emit under 785 nm. When the 785 nm laser option is selected, an Optilab® dRI concentration detector with compatible LED wavelength is paired to the DAWN.
Lignin samples are obtained from a range of natural sources and may be processed in many ways, resulting in distinct variation in the types and amounts of fluorophores used for analysis. Their excitation bands may entirely exclude 785 nm, or this wavelength may be in the very tail of the band. In any case, use of a 785 nm laser is highly effective at suppressing fluorescence from polymers such as lignin, and thus is preferred for these applications. Further suppression of fluorescence can be provided by fluorescence blocking filters, as described in the next section.
Benefits: Suppresses or eliminates fluorescence, enabling reliable characterization of fluorescent samples.
Limitations: The 785 nm laser option produces an increase in the system cost. Importantly, the 785 nm DAWN has approximately one third of the standard DAWN MALS sensitivity for non-fluorescing samples, reducing the signal to-noise ratio (SNR).
.jpg)
Figure 2. Excitation (dashed lines) and emission (unbroken lines) spectra of EGFP (green) and Cy5 (blue). EGFP is not excited by radiation of wavelength longer than about 550 nm, while Cy5 is not excited by wavelengths beyond 700 nm. 532-nm excitation of Cy5 would exhibit low fluorescent intensity and a large Stokes shift while 660-nm excitation would exhibit high fluorescent intensity and a negligible Stokes shift.
DLS:
The DynaPro® NanoStar® cuvette-based DLS detector has very similar wavelength options to the DAWN; the standard instrument uses a 660 nm laser, however this can be replaced with a 785 nm laser.
Benefits: Fluorescence suppression or elimination, enabling reliable characterization of fluorescent samples.
Limitations: Results in a reduction in sensitivity for non-fluorescing samples in a similar manner to MALS.
Transitioning to the DynaPro® Plate Reader can further reduce, if not completely prevent, fluorescence for DLS measurements. The DynaPro® Plate Reader uses a near-infrared 830-nm laser, of which only an extremely small number of fluorescent labels or polymers emit under excitation.
Benefits: Eliminates fluorescence without reducing sensitivity of non-fluorescing samples. Additionally, it also maintains all the benefits of automated DLS.
Limitations: The DynaPro Plate Reader offers about 25% less DLS sensitivity than the NanoStar for non-fluorescing samples. Furthermore, it is not compatible with volatile organic solvents that may degrade the microwell plate, corrode the instrument, or cause a spark. A special quartz plate can be used to measure some organic solvents, but only if the sealing tape is compatible with the solvent. The plate reader can also be more costly in comparison to the cuvette-based DLS for customers who do not need automation.
2. Preventing Fluorescent Radiation from Reaching the Detector: Fluorescence Blocking Filters
Even if fluorescence is emitted, it is always subject to the Stokes shift as it occurs at a longer wavelength than scattered light. Wyatt’s fluorescence-blocking filters stop all (or the majority of) the fluorescing photons from reaching the detector, whilst allowing most of the scattered photons to pass. In most cases, this allows sufficient characterization of molar mass and/or size measurements.
MALS:
Fluorescence-blocking filters can be placed in any of the DAWN’s angular positions. It is recommended to install filters on eight or nine of the DAWN’s 18 detector angles in all the even-numbered positions, leaving the odd-numbered positions open. This sequence of open and filtered angles enables half of the detector to reliably measure non-fluorescing samples, meanwhile the other half can separately measure fluorescing samples.
The standard MALS fluorescence-blocking filters have a relatively wide passband of 20 nm. This allows photons emitted at wavelengths that differ by less than 10 nm from the central laser wavelength to reach the photodiodes. When the Stokes shift is large, i.e. the displacement from the λexc is large, then all the fluorescent photons are outside the passband and are successfully blocked. A narrower passband is required in order to block fluorescence with a smaller Stokes shift.
Benefits: Allows fluorescence suppression or elimination, enabling reliable characterization of fluorescing samples with little impact on the sensitivity of non-fluorescent sample analysis.
Limitations: If the Stokes shift is small then some of the fluorescence is transmitted and taints the intensity measure.
.jpg)
Figure 3. Fluorescence-blocking filters transmit only a narrow band of wavelengths centered on the laser wavelengthλexc.
DLS:
A narrow passband fluorescence blocking filter is available for the Möbius. This filter is an ‘inline’ fluorescence-blocking filter as it is inserted into the optical fiber conveying light to the APD, as opposed to the MALS filters which are installed in the MALS read head. The Möbius’ inline filter has a narrow passband which makes it significantly more effective at blocking low-Stokes-shift fluorescence than MALS filters. Also, the reduction in scattered light reaching the detector is only about 10% despite the narrow passband, preventing any reduction in sensitivity.
Benefits: Fluorescence suppression or elimination, enabling reliable characterization of fluorescence samples with virtually no effect on analysis of non-fluorescing samples.
Limitations: In comparison to 660 nm laser excitation, the 532-nm laser has a higher probability of producing fluorescence in the first place.
3. Light-Scattering Technology Not Adversely Impacted by Fluorescence
The phase of fluorescing photons does not correlate with that of the laser used to excite their emission. When light scattering technique relies on coherence, fluorescing photons cannot affect the results if the detector is not saturated and can respond. PALS is utilized in the Möbius to infer the zeta potential of particles. In PALS, a part of the beam is split off before the flow cell and interferes with the scattered light at the detector array. As the detectors are AC-coupled, they are only sensitive to the difference in frequency between the scattered light and the initial beam. Fluorescing photons have lost all coherence to the incident beam; hence, there is no interference and no difference in frequency, so the detector does not distinguish them.
Wyatt’s Massively-Parallel Phase Analysis Light Scattering (MP-PALS) technology utilizes a high-dynamic-range detector array which, even when subjected to significant fluorescence intensity, does not saturate. This means that, unlike PALS instruments that rely on APDs or photomultipliers, zeta potential analysis in the Möbius is completely unaffected by fluorescence.
Benefits: Provides a measure of zeta potential regardless of fluorescence, in addition to all the benefits of high-sensitivity MPPALS.
Limitations: The forward-scattering geometry of MPPALS does not support zeta potential analysis of turbid samples.
.jpg)
Figure 4. Top: MP-PALS technology implemented in the Möbius. Bottom: While fluorescence increases the overall DC signal in the MP-PALS detectors, they do not saturate and hence there is no effect on the AC-coupled signals.
4. Technologies That Do Not Rely on Light Scattering
Wyatt offers two additional technologies for characterizing molar mass and size that do not rely on light scattering:
Viscometry
Differential viscometers such as the ViscoStar® III are very sensitive to minor changes in solution viscosity arising from dissolved macromolecules. They are used with size-exclusion chromatography to characterize the distribution of intrinsic viscosity [η] of polymers (SECIV). SEC-IV can be used to determine molar mass in two ways. The first is Universal Calibration, which relates elution volume from a column plus the measured intrinsic viscosity to molar mass, assuming that the polymer is a random coil. The second way is with Mark-Houwink-Sakurada (MHS) analysis, which relates molar mass to intrinsic viscosity by an empirical relationship [η]=KMα.
Benefits: Measurements of molar mass are unaffected by fluorescence.
Limitations: Many polymers, such as lignin, are branched, making the assumptions of Universal Calibration analysis erroneous and the results unreliable. Additionally, MHS parameters may not be known, especially for natural polymers that are derived from various sources and processes.
Flow FFF
The basic equation that relates elution of a particle to its diffusion coefficient and hydrodynamic radius in asymmetric-flow field-flow fractionation (AF4) or hollowfiber flow field-flow fractionation (HF5) is derived from first principles. The equation is reliable when the particle undergoes non-ideal interactions with the FFF membrane. The Eclipse® FFF system can be used with just a UV or refractive index detector to infer distributions of macromolecular and nanoparticle sizes from 1-1000 nm in standard mode, and from 1000-10,000 nm in steric elution mode, based just on elution time.
In AF4 or HF5, a method consists of flow conditions and gradients relative to a fixed membrane porosity and spacer size. SCOUT DPS® software utilizes the basic physics of flow-FFF to determine the hydrodynamic radius vs. elution time for a given separation technique. This produces, from the elution profile, the distribution of particle sizes in the analyzed sample.
Benefits: Produces a measure of particle size (hydrodynamic radius) regardless of fluorescence.
Limitations: Fluorescent particles, especially those labeled with a dye, are charged. Assumptions of the Flow-FFF analysis may be invalidated by charged fluorescent particles which consequently interact with the membrane. Therefore, subsequent method development may be required to overcome this interaction.
.jpg)
Figure 5. The SCOUT DPS software determines hydrodynamic size distributions from Eclipse fractograms, using first-principles physics.
A Word About Transmittance
Unavoidably, fluorescent samples also absorb some of the incident laser light before it reaches the detection volume at the center of the flow cell. DLS detectors are sensitive to rapid fluctuations in light intensity rather than overall average intensity and are not overly affected by absorption unless it is very strong and causes heat and convection.
On the other hand, MALS measures average scattered intensity and is affected by absorption. When the sample absorbs, standard MALS analysis misrepresents the amount of light that is scattered; this results in an under-estimation of the apparent molar mass. While the effects of fluorescence (apparent molar mass too high) and absorption (apparent molar mass too low) can partially offset each other, it is not good practice to rely on this bug and turn it into a feature. Wyatt MALS detectors all utilize a Forward Monitor (FM) detector which measures the transmitted beam intensity. When significant absorption occurs, ASTRA can use this FM signal to correct for the change in intensity reaching the detection volume. The absorption is calculated at every point in the chromatogram and the apparent molar mass adjusted to take the absorption into account. Therefore, accurate measurements of these problematic samples are ensured by the combination of FM detection, appropriate laser wavelength and fluorescence blocking filters.
.jpg)
Figure 6. Forward Monitor signal (blue) exhibits a dip due to absorption by the sample, coinciding with peak scattering (red).
The FM correction is important for many different samples that absorb at the laser wavelength, even if they are non-fluorescing. For instance, heme-containing proteins absorb the red laser; without this feature, the measured molar mass would be significantly too low. Thus, this shows how the additional transmittance detector is vital for characterizing a variety of macromolecules.
Is Fluorescence Giving You Bad Data?
There are several patterns of behavior in MALS and DLS that can indicate the presence of fluorescence.
The two primary symptoms in MALS are:
1. Absorption, identified as a dip in the FM signal that coincides with the peak.
2. Abnormally high molar mass calculated by light scattering, relative to the elution position in the chromatogram.
The two primary symptoms in DLS are:
1. Flat ACF, which looks like a pure buffer or very small particles.
2. High count rate, in the tens of millions.
If such symptoms occur, Wyatt recommends checking your sample in a spectrofluorimeter to determine the excitation and emission spectra.
Conclusion
Despite the challenges of performing light scattering measurements on fluorescent samples, Wyatt Technology offers solutions for reliably characterizing these materials. Such solutions include preventing the excitation of fluorescence with a longer wavelength laser and preventing fluorescent radiation from reaching the detector with fluorescence blocking filters. Alternatively, Wyatt also offers light scattering technologies that are not impacted by fluorescence, or non-light scattering techniques that can conduct measures of absolute molar mass and size.
.jpg)
Acknowledgements
The conjugated nanoparticle samples were kindly provided by the lab of Prof. Ronit Satchi-Fainero at the Tel-Aviv University Faculty of Medicine.
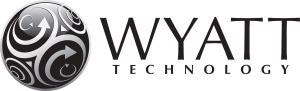
This information has been sourced, reviewed and adapted from materials provided by Wyatt Technology.
For more information on this source, please visit Wyatt Technology.