Nanobiotechnology involves the application of nanoscale technologies to the field of biological and life science. Recently, researchers have investigated whether the size of gold nanoparticles (AuNPs) influences their antibacterial abilities on three strains of Streptococcus, a common dental bacteria.
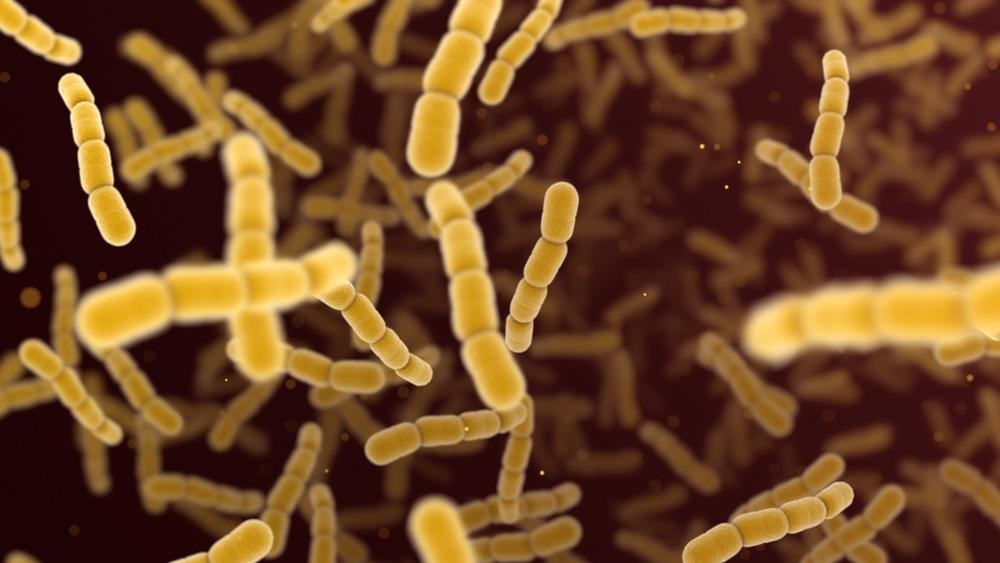
Image Credit: Jezper/Shutterstock.com
Dental caries are the commonly seen human infectious disease, involving various pathogenic factors and microbes like Streptococcus salivarius (S. salivarius), Streptococcus mutans (S. mutans), and Streptococcus sanguinis (S. sanguinis), salivary related disorders, and individual diet.
Over the years, various preventive methods have been introduced for dental caries, such as metals, because of their antibacterial properties.
A metal’s antibacterial properties are thought to be affected by how big its surface is; larger surface areas of metal nanoparticles result in more potent interactions with various other molecules.
A study published in the Journal of Dentistry has analyzed varying sizes of silver, zinc oxide and gold nanoparticles against strains of Streptococcus. Average sizes of 25, 125 and 80 nm were explored.
Methodology
This study had 75 children between the ages of three and five years participating for six months. Children’s dental caries were assessed utilizing bitewing radiographs and dental explorers.
Using a sterile toothpick, 75 specimens of dental plaque with dental caries were obtained. Moreover, unstimulated saliva from the sublingual region was collected using a sterile cotton swab.
The cultures were incubated anaerobically and S. sanguinis colonies were selected. The prototype strain of S. mutans (ATCC 25175), S. sanguinis (ATCC 10556), and S. salivarius (ATCC9759) were employed as standard species.
Polymerase chain reaction (PCR) was utilized for biochemical tests confirmation (respectively Figures 1, 2, and 3).
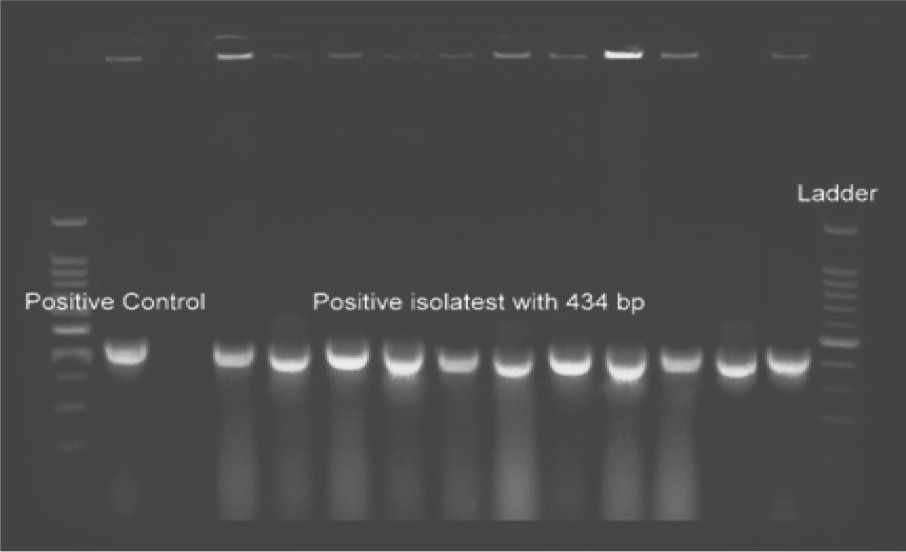
Figure 1. Image Credit: Lavaee, et al., (2021)
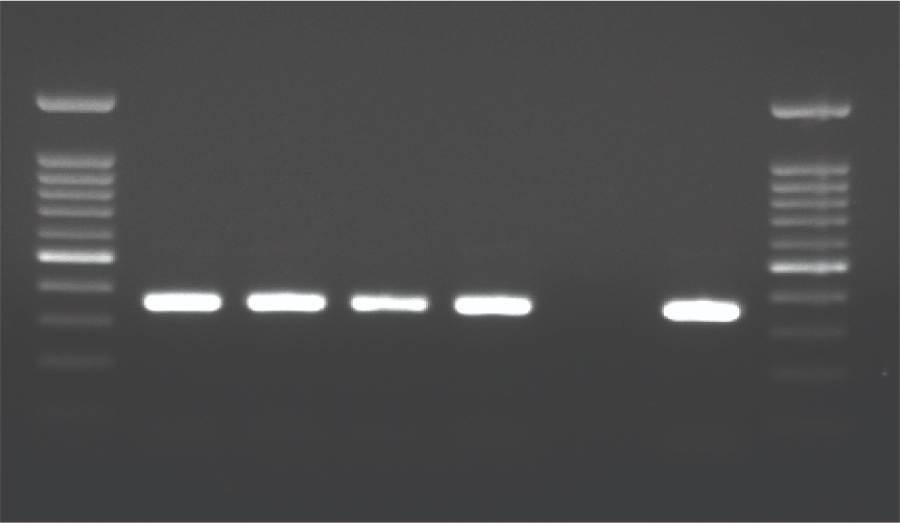
Figure 2. Image Credit: Lavaee, et al., (2021)
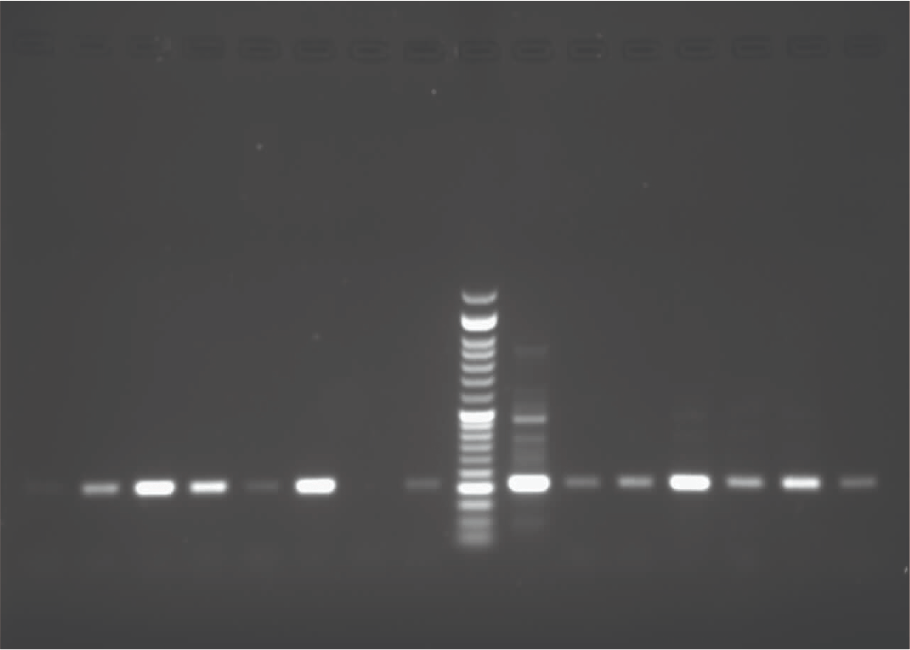
Figure 3. Image Credit: Lavaee, et al., (2021)
The gradient system confirmed the annealing temperature. PCR amplification of isolated S. mutans, S. sanguinis, and S. salivarius species are shown in Figures 1, 2, and 3. The antibacterial assessment was carried out on clinically isolated bacteria and standard species.
A water-based solution of nanoparticles was prepared, and the size distribution of the nanoparticles was confirmed by ultraviolet-visible spectroscopy and a particle size analyzer (see Figure 4). Mean size ranged from 25 to 90 nm for nanoparticles.
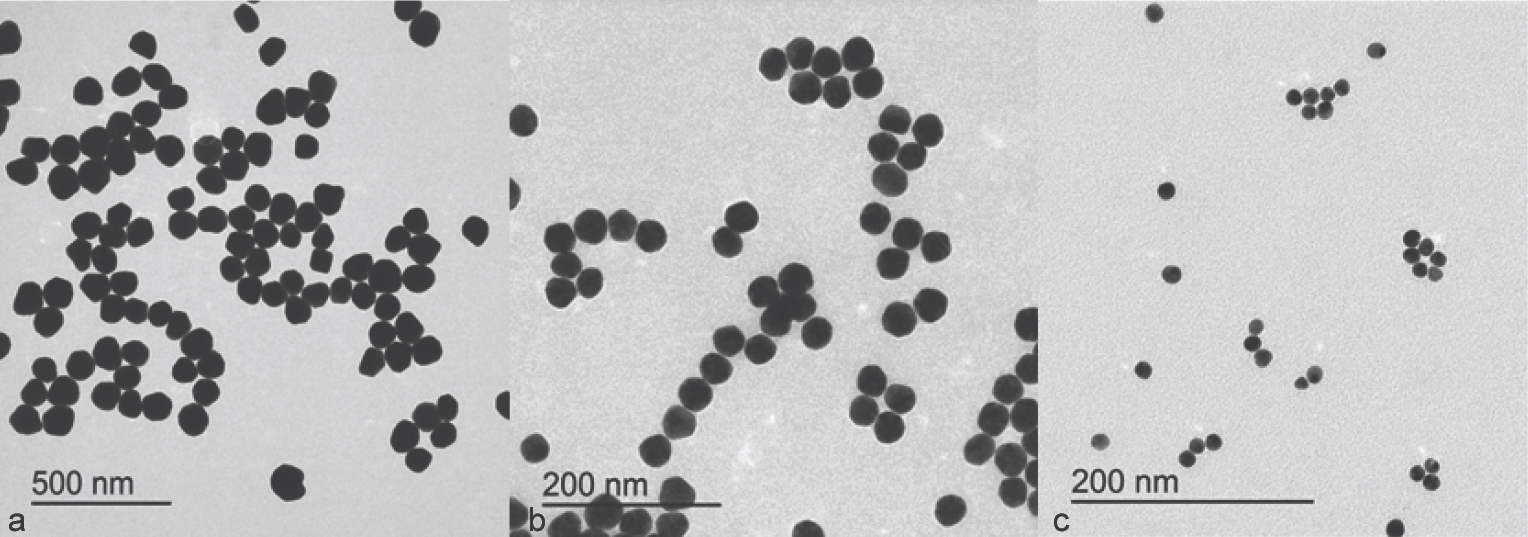
Figure 4. Image Credit: Lavaee, et al., (2021)
Colloidal solutions of nanoparticles with an initial concentration of 500 µg/mL, were sterilized, and the minimum bactericidal concentration (MBC) and minimum inhibitory concentration (MIC) were determined.
Evaluation of the MIC and MBC values of AuNPs (gold nanoparticles) various sizes against standard species of S. sanguinis, S. mutans, and S. salivarius were also performed, data were analyzed by SPSS version 18. To compare the MIC and MBC values, ANOVA and Post-hoc Tukey were used.
Results
Tables 1 and 2 list the MBC and MIC of AuNPs against patient-isolated bacteria and standard species.
The team noted that the MIC and MBC of different sizes of AuNPs against different patient-isolated bacteria and standard species were statistically different.
Table 1. Minimum inhibitory and minimum bactericidal concentration mean of gold nanoparticles against patient-derived S. mutans, S. salivarius, and S. sanguinis. Source: Lavaee, et al., (2021)
Size (nm) bacteria |
MIC mean (µg/ml) |
MBC mean (µg/ml) |
25 |
S.mutans |
1.73±1.23 |
4.05±2.68 |
S.sanguinis |
3.17±1.50 |
6.46±2.98 |
S.salivarius |
2.86±1.58 |
6.09±3.15 |
Total |
2.51±1.55 |
5.41±3.09 |
60 |
S.mutans |
91.61±46.39 |
184.65±91.37 |
S.sanguinis |
148.21±64.46 |
289.28±124.15 |
S.salivarius |
119.31±63.20 |
242.42±124.76 |
Total |
117.46±61.82 |
234.37±119.97 |
90 |
S.mutans |
232.95±124.53 |
217.26±236.59 |
S.sanguinis |
353.57±130.34 |
329.53±326.91 |
S.salivarius |
329.54±124.28 |
302.53±314.42 |
Total |
299.10±136.42 |
277.47±294.03 |
Table 2. Minimum inhibitory and minimum bactericidal concentration mean of gold nanoparticles against standard S. mutans, S. salivarius, and S. sanguinis. Source: Lavaee, et al., (2021)
AuNPs |
S. salivarius
(ATCC 9759) |
S. sanguinis
(ATCC10556) |
S. mutans
(ATCC 25175) |
MBC (µg/ml) |
MIC (µg/ml) |
MBC (µg/ml) |
MIC (µg/ml) |
MBC (µg/ml) |
MIC (µg/ml) |
25 nm |
1.95 |
0.97 |
3.9 |
1.95 |
7.81 |
1.95 |
60 nm |
125 |
62.5 |
125 |
62.5 |
250 |
125 |
90 nm |
500 |
250 |
1000 |
500 |
500 |
250 |
Chlorhexidine |
50 |
50 |
50 |
25 |
50 |
50 |
The MBC and MIC of chlorhexidine against the three patient-isolated and standard species of streptococcus (see Table 2), were higher than values registered for 25 nm AuNPs. The MBC and MIC values of AuNPs against patient-isolated S. sanguinis, S. mutans, and S. salivarius are illustrated in Figures 5 and 6, respectively.
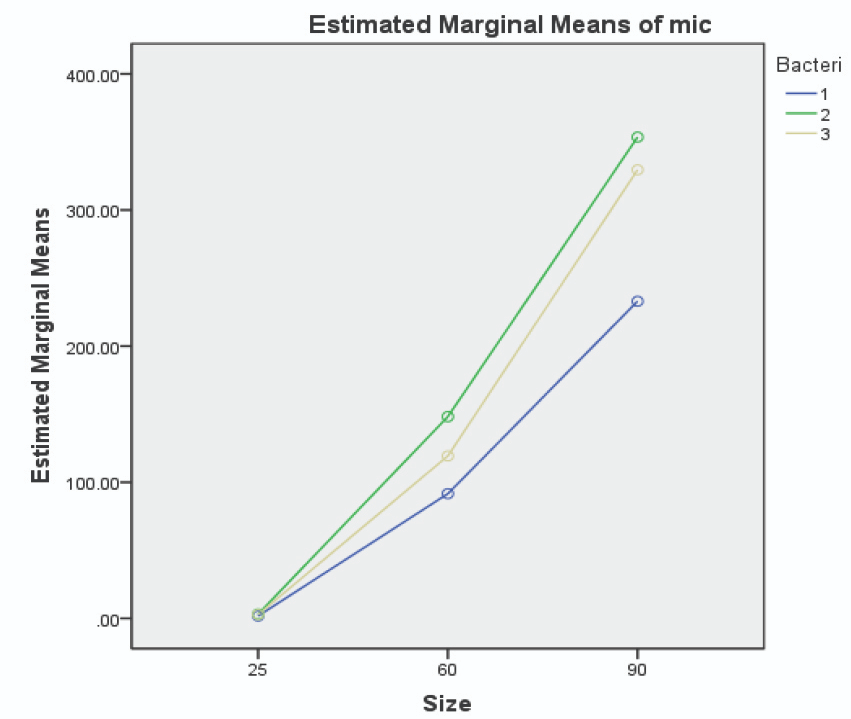
Figure 5. Image Credit: Lavaee, et al., (2021)
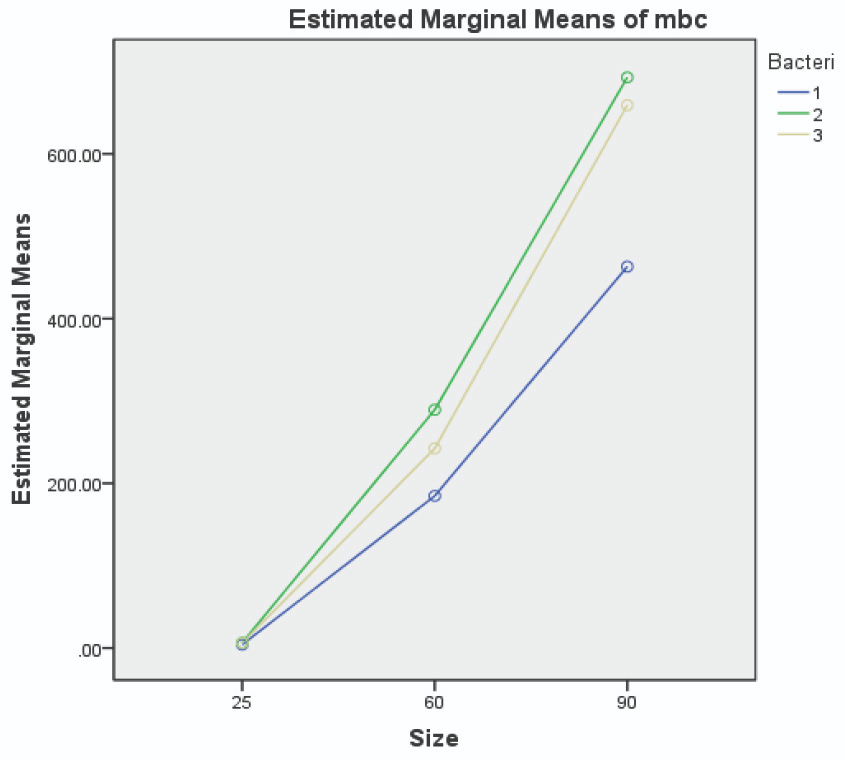
Figure 6. Image Credit: Lavaee, et al., (2021)
Smaller AuNPs were more potent antibacterial agents, and 25 nm AuNPs had the lowest MIC and MBC against both patient-isolated and standard bacteria. In many studies, nanoparticles have demonstrated a wide range of antibacterial activity against different bacteria, both gram-positive and gram-negative.
Nanoparticles can attach to the cell membrane and disrupt its permeability. By reducing the size of nanoparticles, more gold ions can be released, and antibacterial properties are enhanced.
Anaerobic bacteria may be prevented from releasing nanoparticles due to insufficient air; therefore, the different release of nanoparticle ions makes the difference in antimicrobial potencies for aerobic and anaerobic bacteria. The antibacterial effect of nanoparticles is related to their concentration.
However, almost all studies support the size-dependent antimicrobial effect of nanoparticles in a similar manner; as the size of nanoparticles decreases, the antimicrobial effect increases.
Conclusion
The current study evaluated the antibacterial potency of three different sizes of AuNPs on both clinically isolated and standard species.
Here, a correlation was shown between the size of AuNPs and their antibacterial activity. As the size of AuNPs decreases, the antibacterial properties improve. The patient-isolated bacteria are more defiant to the antibacterial effect of AuNPs.
Continue reading: Improving Dental Restorative Materials Using Nanotechnology.
Journal Reference:
Lavaee, F., Ranjbar, Z., Modarezi, F., Keshavarz, F. (2021) The Effect of Gold Nano Particles with Different Sizes on Streptococcus Species. Journal of Dentistry, 4(73), pp. 235–242. Available online: https://dentjods.sums.ac.ir/article_47735.html.
References and Further Reading
- Abiodun-Solanke, I. M. F., et al. (2014) Nanotechnology and its Application in Dentistry. Annals of Medical and Health Science Research, 4(3), pp. S171–S177.
- Ozak, S T & Ozkan, P (2013) Nanotechnology and dentistry. European Journal of Dentistry, 7, pp. 145–151.
- MubarakAli, D., et al. (2011) Plant extract mediated synthesis of silver and gold nanoparticles and its antibacterial activity against clinically isolated pathogens. Colloids and Surfaces B: Biointerfaces, 85, pp. 360–365. doi.org/10.1016/j.colsurfb.2011.03.009.
- Annamalai, A., et al. (2013) Green synthesis, characterization and antimicrobial activity of Au NPs using Euphorbia hirta L. leaf extract. Colloids and Surfaces B Biointerfaces, 108, pp. 60–65. doi.org/10.1016/j.colsurfb.2013.02.012.
- Hernández-Sierra, J. F., et al. (2008) The antimicrobial sensitivity of Streptococcus mutans to nanoparticles of silver, zinc oxide and gold. Nanomedicine, 4, pp. 237–240. doi.org/10.1016/j.nano.2008.04.005.
- Ghapanchi, J., et al. (2015) The antibacterial effect of four mouthwashes against Streptococcus mutans and Escherichia coli. Journal of Pakistan Medical Association, 65(4), pp. 350–353.
- Lavaee, F., et al. (2016) Antimicrobial and antibiofilm activity of silver, titanium dioxide and iron nano particles. American Journal of Dentistry, 29, pp. 315–320.
- Behbahan, G. F., et al. (2016) Antimicrobial activities of Gold nanoparticles against Salmonella typhimurium. Advanced Herbal Medicine, 2, pp. 26–30.
- Martínez-Castañón, G. A., et al. (2008) Synthesis and antibacterial activity of silver nanoparticles with different sizes. Journal of Nanoparticle Research, 10, pp. 1343–1348. doi.org/10.1007/s11051-008-9428-6.
- Zhou, Y., et al. (2012) Antibacterial activities of gold and silver nanoparticles against Escherichia coli and bacillus Calmette-Guérin. Journal of Nanobiotechnology, 10, p. 19. doi.org/10.1186/1477-3155-10-19.
- Wenzel, A (1998) Digital radiography and caries diagnosis. Dentomaxillofacial Radiology, 27, pp. 3–11. doi.org/10.1038/sj.dmfr.4600321.
- Caufield, P. W., et al. (2000) Natural History of Streptococcus sanguinis in the Oral Cavity of Infants: Evidence for a Discrete Window of Infectivity. Infection Immunity, 68, pp. 4018–4023. doi.org/10.1128%2Fiai.68.7.4018-4023.2000.
- Loesche, W. J., et al. (1975) Association of Streptococcus mutants with human dental decay. Infection Immunity, 11, pp. 1252–1260.
- Marsh, P., et al. (1989) A microbiological study of early caries of approximal surfaces in schoolchildren. Journal of Dental Research, 68, pp. 1151–1154. doi.org/10.1177/00220345890680070301.
- Jordan, H. V., et al. (1987) Simplified diagnostic system for cultural detection and enumeration of Streptococcus mutans. Journal of Dental Research, 66, pp. 57–61. doi.org/10.1177/00220345870660011201.
- Garnier, F., et al. (1997) Identification of clinically relevant viridans group streptococci to the species level by PCR. Journal of Clinical Microbiology, 35, pp. 2337–2341.
- Najjar, M. B., et al. (2009) Natural antimicrobials ε-poly-l-lysine and Nisin A for control of oral microflora. Probiotics Antimicrobial Proteins, 1, p. 143. doi.org/10.1007/s12602-009-9020-0.
- Agnihotri, S., et al. (2014) Size-controlled silver nanoparticles synthesized over the range 5–100 nm using the same protocol and their antibacterial efficacy. RSC Advances, 4, 3974–3983. doi.org/10.1039/C3RA44507K.
- Espinosa-Cristóbal, L., et al. (2009) Antibacterial effect of silver nanoparticles against Streptococcus mutans. Materials Letters, 63, pp. 2603–2606. doi.org/10.1016/j.matlet.2009.09.018.
- Yamamoto, O (2001) Influence of particle size on the antibacterial activity of zinc oxide. Solid-State Chemistry, 3, pp. 643–646. doi.org/10.1016/S1466-6049(01)00197-0.
- Pal, S., et al. (2007) Does the Antibacterial Activity of Silver Nanoparticles Depend on the Shape of the Nanoparticle? A Study of the Gram-Negative Bacterium Escherichia coli. Applied and Environmental Microbiology, 73, pp. 1712–1720. doi.org/10.1128/aem.02218-06.
- Raghupathi, K. R., et al. (2011) Size-dependent bacterial growth inhibition and mechanism of antibacterial activity of zinc oxide nanoparticles. Langmuir, 27, pp. 4020–4028. doi.org/10.1021/la104825u.
- Nagarajan, P & Vijayaraghava, R (2008) Enhanced bioactivity of ZnO nanoparticles—an antimicrobial study. Science and Technology of Advanced Materials, 9, p. 035004. doi.org/10.1088/1468-6996/9/3/035004.
- Rai, M., et al. (2009) Silver nanoparticles as a new generation of antimicrobials. Biotechnology advances, 27, pp. 76–83. doi.org/10.1016/j.biotechadv.2008.09.002.
- Lok, C. N., et al. (2006) Proteomic analysis of the mode of antibacterial action of silver nanoparticles. Journal of Proteome Research, 5, pp. 916–924. doi.org/10.1021/pr0504079.
- Holt, K. B., et al. (2005) Interaction of silver(I) ions with the respiratory chain of Escherichia coli: an electrochemical and scanning electrochemical microscopy study of the antimicrobial mechanism of micromolar Ag+. Biochemistry, 44, pp. 13214–13223. doi.org/10.1021/bi0508542.
- Eby, D. M., et al. (2009) Hybrid antimicrobial enzyme and silver nanoparticle coatings for medical instruments. ACS Applied Materials Interfaces, pp. 11553–1560. doi.org/10.1021/am9002155.
- Goodman, C. M., et al. (2004) Toxicity of gold nanoparticles functionalized with cationic and anionic side chains. Bioconjugate Chemistry, 15, pp. 897–900. doi.org/10.1021/bc049951i.
- Marini, M., et al. (2007) Antibacterial activity of plastics coated with silver-doped organic-inorganic hybrid coatings prepared by sol-gel processes. Biomacromolecules, 8, pp. 1246–1254. doi.org/10.1021/bm060721b.
- Yang, H., et al. (2009) Comparative study of cytotoxicity, oxidative stress and genotoxicity induced by four typical nanomaterials: the role of particle size, shape and composition. Journal of Applied Toxicology, 29, pp. 69–78. doi.org/10.1002/jat.1385.
- Xia, T., et al. (2008) Comparison of the Mechanism of Toxicity of Zinc Oxide and Cerium Oxide Nanoparticles Based on Dissolution and Oxidative Stress Properties. ACS Nano, 2, pp. 2121–2234. doi.org/10.1021/nn800511k.
- Zhang, L., et al. (2007) Investigation into the antibacterial behaviour of suspensions of ZnO nanoparticles (ZnO nanofluids). Journal of Nanoparticle Research, 9, pp. 479–489. doi.org/10.1007/s11051-006-9150-1.
- Brayner, R., et al. (2003) Toxicological impact studies based on Escherichia coli bacteria in ultrafine ZnO nanoparticles colloidal medium. Nano Letters, 6, pp. 866–870. doi.org/10.1021/nl052326h.
- Roselli, M., et al. (2003) Zinc oxide protects cultured enterocytes from the damage induced by Escherichia coli. The Journal of Nutrition, 133, pp. 4077–4082. doi.org/10.1093/jn/133.12.4077.
- Lu, Z., et al. (2013) Size-dependent antibacterial activities of silver nanoparticles against oral anaerobic pathogenic bacteria. Journal of Materials Science: Materials in Medicine, 24, pp. 1465–14671. doi.org/10.1007/s10856-013-4894-5.
- Thiel, J., et al. (2007) Antibacterial properties of silver-doped Titania. Small, 3, pp. 799–803. doi.org/10.1002/smll.200600481.