Much research has been conducted on two-dimensional (2D) materials due to their unique mechanical, optical, and electrical characteristics. Ever since the peculiar physical characteristics of graphene were discovered in 2004, research teams worldwide have concentrated on the exploration, creation, and utilization of two-dimensional materials.
This rush towards 2D materials included the study of binary compounds BN (901349, 901410), transition metal dichalcogenides (TMDs) (901867, 901187, 902462, 903841), oxides, and single elemental structures like phosphorene (902896), stanene, silicene, germanene (906026), etc.
2011 a novel family of conductive 2D carbides, nitrides, and carbonitrides, known as MXenes, was discovered. MXenes are one of the largest classes of 2D materials, with more than 30 types reported (Figure 1) and hundreds computationally studied in-silico. Moreover, this family could have thousands of additional members if solid solutions are included.
MXenes tend to come in the form of Mn+1XnTx, where M is an early transition element (Ti, V, Nb, etc.) and X is C and/or N, with n ranging from 1–4. Tx represents the surface terminations (typically -O, -OH, and -F), with n+1 layers of M covering n layers of X in the arrangement of [MX]nM.
These hydrophilic, conductive 2D ceramics are made by selectively etching the MAX phases (910740, 910767, 910775, 910759, 910821, 910708) of their precursor materials top-down. The resulting Mn+1AXn is the typical form of these MXenes; however, some are also made from M2A2X and Mn+1AxXn+x.
The synthesis of MXenes is also scalable, with no change in the properties as the batch size is increased. In these cases, A is primarily Al, but Si and Ga have also been used.
Multiple forms of MXenes exist: ordered double transition metal MXenes (e.g., Mo2Ti2C3Tx, Mo2TiC2Tx, Cr2TiC2Tx), solid solution MXenes (e.g., Ti2-yVyCTx, Mo4-yVyC3Tx, Ti2-yNbyCTx), single metal element structures (e.g., Ti3C2Tx, Ti2CTx, V2CTx, etc.), ordered divacancy MXenes (Mo1.33CTx, W1.33CTx, etc.).
The MXene family is quite diverse, with materials that can be tuned through a variety of approaches, including modification of the number of atomic layers (n), changing the M or X elements, adjusting the surface chemistry (Tx) through post-treatment or during synthesis, size selection of MXenes, and intercalation of different species into the structure, among multiple other approaches. Such tunability and its range of properties offer a diverse number of applications (Figure 2). Whilst MXenes were initially used as materials for energy storage, in 2016 we observed they had been explored in high-gen sensors, biomedical devices, structural, catalysis, water treatment, photodetectors and more.
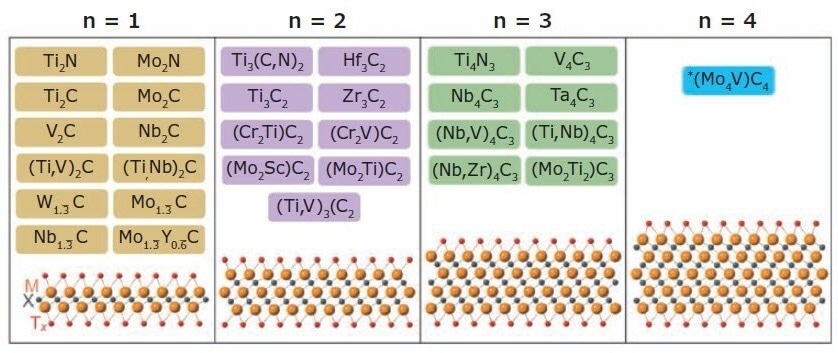
Figure 1. MXene compositions reported to date. MXenes have the general structure Mn+1XnTx where M is an early transition metal (Ti, V, Nb, etc.), X is C and/or N, Tx are the surface terminations (typically -O, -OH, -Cl and -F), and n = 1–4. MXenes discovered to date include mono-M MXenes, ordered double-transition metal MXenes, solid-solution MXenes, and ordered divacancy MXenes. Image Credit: Merck
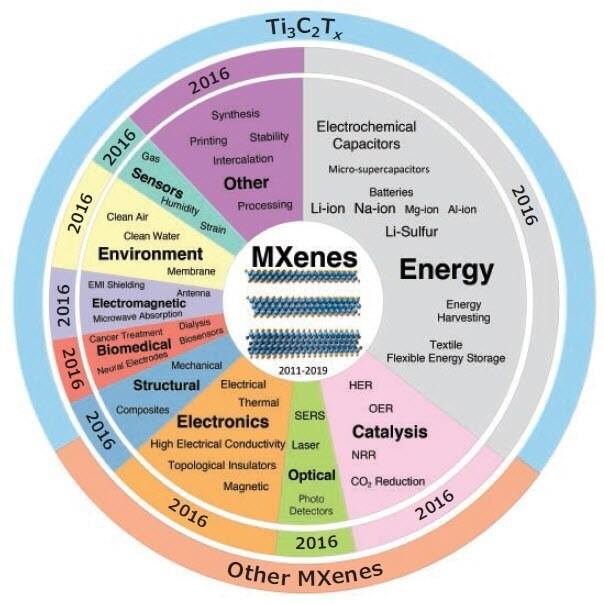
Figure 2. Applications and properties of MXenes that have been explored to date. The center pie chart shows the ratio of publications in each application/ property of MXenes with respect to the total number of publications on the “MXene” topic from 2011 to February 2019 based on Web of Science. The middle pie chart ring, with similar color to the center one, shows the starting year for exploration of each application/property of MXenes. There might be one or two papers published before some of the mentioned years; we considered a year with a few important publications as the starting year for each slice. The outer ring shows the ratio of publications only on Ti3C2Tx MXene versus the publications on all MXene compositions (M2XTx, M3X2Tx, M4X3Tx), and M5X4Tx). Image Credit: Merck
Energy Storage and MXenes
A lot of research has gone into using different MXenes as materials for energy storage. Ti3C2Tx, for example, was shown to have a volumetric capacitance of up to 1,500 F cm-3. In light of this, it is anticipated that thinner (M2XTx) MXenes will have a higher theoretical gravimetric capacity.
Within energy storage devices, MXenes have been used as electrodes in electrochemical capacitors, micro-supercapacitors, and batteries within energy storage devices, using Li-, Na-, Mg-, Al-, and other chemistries. A wide range of electrolytes, including ionic liquids and non-aqueous organic solutions (e.g., DMSO, PC, acetonitrile (ACN), etc.)-based solutions, have been used, including aqueous solutions (e.g., H2SO4, Li2SO4, KOH, etc.).
The usable voltage range falls between 0.5 to 3.0 V, depending on the electrolyte system that is being used. Due to the large number of inherent chemistries available to MXenes, a lot of research has been carried out to explore the charge storage mechanisms of various MXenes, as well as how to optimize the materials further.
MXenes typically have a pseudocapacitive storage mechanism; the ions penetrate between MXene sheets, interacting with the surface terminations on the basal plane. The MXene interlayer spacing can be adjusted to accommodate the size of the intercalating ion based on the ions that are used.
Lastly, MXenes exhibit outstanding cyclability; after 10,000 cycles for Ti3C2Tx in aqueous electrolytes, there was no discernible change in capacitance.
Additionally, using metallically conductive MXenes as binders and current collectors in energy storage devices has proven to be very promising. Although a significant portion of MXene research has focused on energy storage capabilities, a variety of other applications have also been explored.
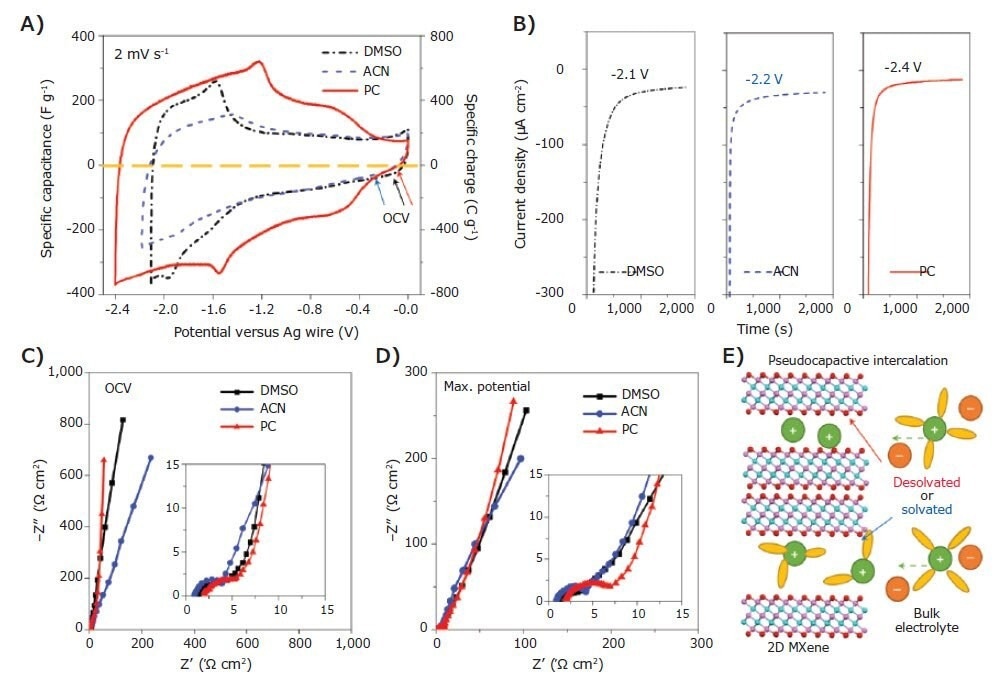
Figure 3. Macroporous Ti3C2Tx electrode with 1 M LiTFSI in DMSO, ACN and PC organic electrolytes. A), CV curves. The OCVs (marked by arrows) are −0.13 V (black), −0.32 V (blue) and −0.12 V (red) versus AgCI for DMSO, ACN and PC-based electrolytes, respectively. B), Chronoamperometry data collected at the applied maximum potentials. C), EIS data collected at the OCV. D), EIS data collected at the maximum negative potential versus AgCI. The insets in C and D show the magnified curves in the high-frequency range; they use the same units as in C and D. E), Schematic of a supercapacitor using 2D MXene (pink, Ti; cyan, C; red, O) as negative electrode with solvated or desolvated states. Legend for the electrolyte: green, cation; orange, anion; yellow, solvent molecule. Image Credit: Merck
MXene Phases
Source: Merck
MAX Phases
Source: Merck