High resolution, swift, harmless, and quantifiable characterizing techniques are required to design substances with customized characteristics at the nanometer scale or to comprehend the link between mechanical characteristics and cell functionality.
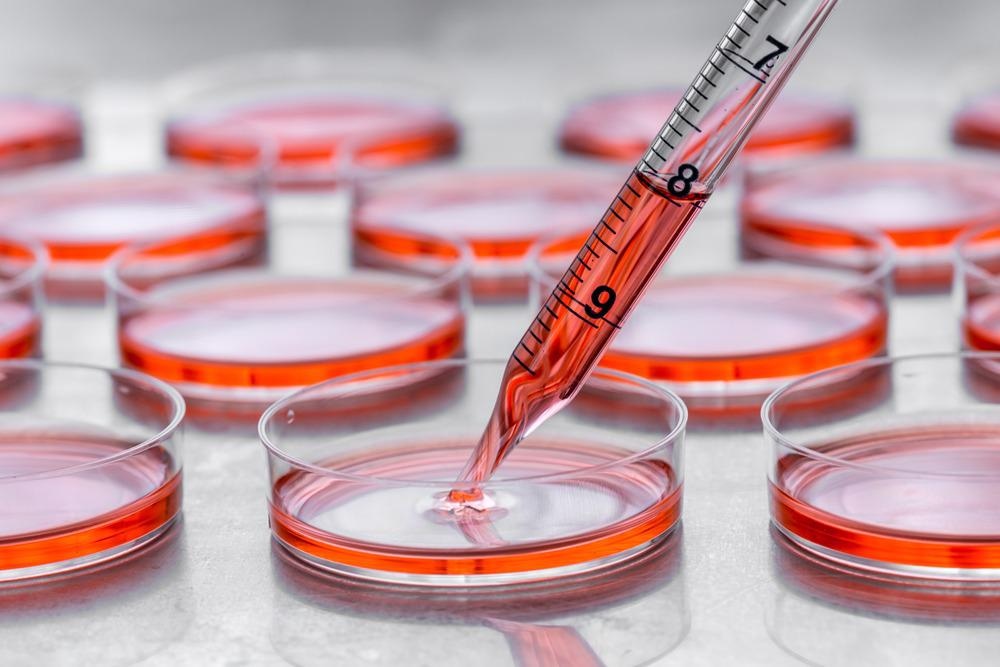
Image Credit: Hakat/Shutterstock.com
Atomic force microscopy (AFM) has significantly improved our knowledge of soft substances and their mechanical, biomolecular, and physiochemical interactions. One of the important successes of AFM is measuring the mechanical characteristics of heterogeneous surfaces at elevated spatial resolutions.
The atomic force microscope (AFM) was created to address a fundamental limitation of scanning tunneling microscopy (STM): it can only scan conductive or semiconductive materials. The AFM has the benefit of being able to image practically any material, such as ceramics, polymers, composite materials, glass, and organic matter.
Where is AFM Needed?
The development of AFM has been influenced by several conditions. In materials engineering, the construction of heterogenized products entails mapping the mechanical characteristics of the resultant junctions with fine spatial resolution and mathematical precision.
Time-resolved mapping of physical engagements is required in mechanobiology to properly comprehend cell development and proliferation. Finally, the ability of AFM to provide high-resolution pictures of a wide range of solid–liquid interfaces necessitates rapid and reliable techniques for mapping free edge forces.
How AFM Works
Nanomechanical force spectroscopy draws on various technical disciplines, from applied physics and physiochemical concepts to biomolecular studies to mechanical engineering. It also includes a wide range of objectives across the academic and industrial sphere. Although these variables have resulted in an abundance of experimentation methodologies and use, they have also led to the formation of a muddled domain.
As a mechanical kind of microscope, AFM converts the interactive force that acts between a sharpened tip and a test piece into cantilever-tip transducer movement. To measure the tip-sample contact, atomic forces are utilized rather than the quantum mechanical tunneling effect.
AFM examines the mechanical characteristics of a sample in one of two ways: either by measuring the interactive force in terms of the tip–sample separation or by recording the influence of the interactive force on certain oscillatory criteria of the tip.
Over two decades ago, the AFM's capacity to create mechanical property profiles of soft substances was identified. One of AFM's earliest successes was the nanoscale analysis of polymeric surfaces in their original condition.
Ever since, a wide range of approaches for characterizing soft substances has been presented, refined, and implemented. Some of the early approaches, like force–volume and AFM phase-imaging, are still being developed, whereas other methods' applicability has waned.
The Various Nanomechanical Mapping Methods
Modern AFM-based technologies can record the mechanical characteristics of soft substances at extremely high (<10 nm) or high (10–100 nm) pixel density. Soft substances are roughly characterized as having a Young's Modulus ranging from 100 Pa to 10 GPa, and include cells, proteins, liposomes, polymers, and biopolymers.
A sophisticated nanomechanical mapping approach must have these characteristics: excellent spatial resolution; quantitative reliability; independence from tip characteristics; rapid data collection (at least one frame per second); and minimization of crosstalk among topographic and material characteristics.
To obtain mappings of mechanical characteristics with high spatial resolution, many AFM-based approaches have been developed. Initially, the approaches were categorized based on the AFM mode employed to create a topographical picture, which might be dynamic (resonance on), or quasi-static (resonance off). AFM phase-imaging force–volume, bi-modal AFM, and contact resonance are the most often utilized nanomechanical modeling techniques.
Current Limitations of AFM
Living cells, particularly eukaryotes, present certain hurdles to an AFM's image processing and modeling abilities. Currently, the best images of eukaryotic cells acquired by AFM have a spatial resolution of 100 nm.
Compared to the spatial resolution reached on other biomolecules such as proteins (1 nm) or protein fibers, these results are small. Determining the Young's Modulus of a cell with precision is particularly difficult since various variables add to measurement inaccuracy.
To address and finally overcome these challenges, it is necessary to first understand the function of the primary elements that affect the imaging and modeling mechanical characteristics.
For the operational and composition assessment of biological samples on-site, optical microscopy is still the technique of choice. Images from optical microscopy enable broad viewing ranges, accessibility to organelles deep within the cell, and molecular tagging.
Potential Avenues for Progress
The integration of nanomechanical mapping with various optical microscopy approaches could be very advantageous for cellular imaging. Immunofluorescence might be used at the target tissues to direct the AFM tip to particular material parts. This combination connects characteristic mechanical evaluation to biochemical makeup. At the cellular level, the combination of high-resolution imaging with nanomechanical mapping would provide novel insights into cell dynamics and functioning.
Big data analytics and machine learning techniques' promise in AFM and force spectroscopy is still mostly unrealized. Huge data files will be generated by rapid nanomechanical mapping, necessitating efficient processing methods.
Simultaneously, numerous technical and medicinal applications will need the automation of AFM imaging. Automation, machine learning, and other computational techniques are likely to contribute to the discipline.
On a Final Note
The advent of nanotechnology prompted the creation of the very first nanomechanical mapping technologies. Today, several domains such as mechanobiology, smart material creation, power storing, nanoscale medicine, and clinical diagnostics offer motives to improve the competencies of nanomechanical force spectroscopy.
Improved theoretical frameworks and improved data analytics methods are likely to be introduced into the field in the coming years. Simultaneously, new hardware and designs will enable high-speed nanomechanical mapping.
Continue reading: 2D Nanomaterials in Cancer Nanotheranostics.
References and Further Reading
Gavara, N., 2018. AFM-Based Nanomechanics. [online] Wiley Analytical Science. https://analyticalscience.wiley.com/do/10.1002/imaging.6333
Eaton, P., & West, P. (2010). Atomic force microscopy. Oxford university press.
Garcia, R. (2020). Nanomechanical mapping of soft materials with the atomic force microscope: methods, theory and applications. Chemical Society Reviews(16). Available at: https://doi.org/10.1039/D0CS00318B
Disclaimer: The views expressed here are those of the author expressed in their private capacity and do not necessarily represent the views of AZoM.com Limited T/A AZoNetwork the owner and operator of this website. This disclaimer forms part of the Terms and conditions of use of this website.