Semiconductor lasers are versatile, efficient and portable solid-state lasers that have seen remarkable power, speed, and size advancements in the last few decades. With the advent of nanotechnology, researchers are now exploring the development of semiconductor nanolasers, which offer potential applications in communication, high-speed displays, material processing and biomedical applications.
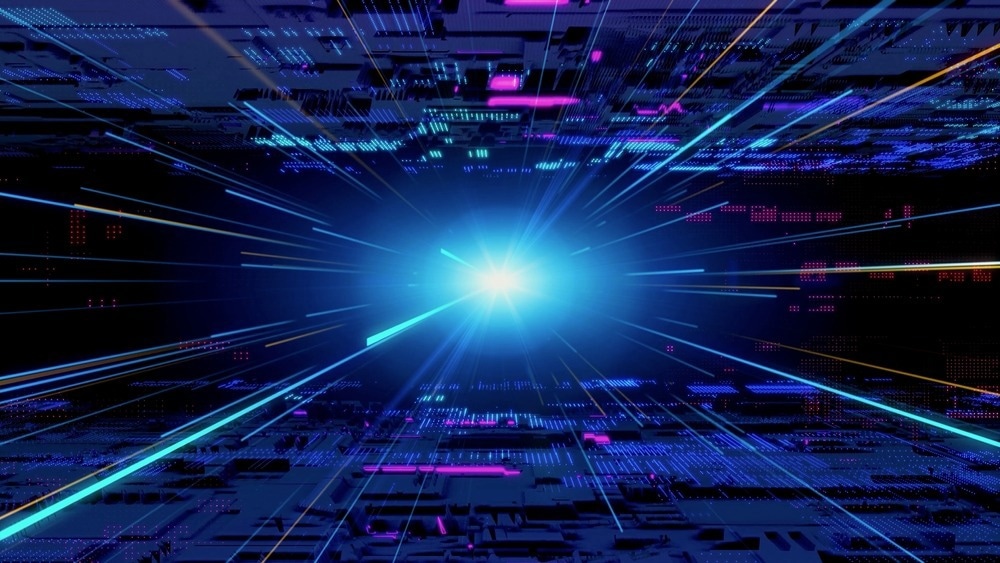
Image Credit: metamorworks/Shutterstock.com
Semiconductor Lasers
Semiconductor lasers are small solid-state lasers that use a semiconductor as an active medium to amplify light through stimulated emission at inter-band transitions under high electron density in the conduction band.
They are highly efficient and compact lasers, typically less than one millimeter in size, with commercial models emitting wavelengths ranging from 600 nanometers in the red spectrum to 1.6 micrometers in the near-infrared range.
Advantages of Semiconductor Lasers
Semiconductor lasers offer numerous advantages, including their small size, high reliability, lightweight, low power consumption, and long lifespan. In addition, they operate on the low-voltage constant current mode, which reduces the likelihood of power failures, ensures safe operation, and lowers maintenance costs.
Working Principle
The radiative recombination of electron-hole pairs in the active medium generates optical emission from semiconductor lasers. During this process, electrons from the conduction band fill the holes in the valence band, releasing surplus energy as photons. The emitted photons then stimulate further electron-hole recombination, resulting in stimulated emission and laser light production.
The semiconductor material’s bandgap (the energy difference between the valence and conduction bands) determines the laser frequency and energy.
Applications
Due to their mass-production capacity and compact size, semiconductor lasers are the most popular lasers with a wide range of applications in optical networking and communication, laser printing, optical data storage, barcode scanning, material processing, and biomedical and forensic diagnostics.
Integrated Optical Interconnects
Semiconductor lasers are currently the most feasible light source for fiber optic communication systems, making them an essential component of modern communication technology.
As global internet traffic grows exponentially, reducing energy consumption in data communications is crucial for sustainability. While edge-emitting lasers have long been used for long-haul optical links, semiconductor lasers are now preferred for short-distance optical interconnects.
This is because semiconductor lasers are smaller and use less energy per bit, making them more efficient.
Semiconductor Laser-Interference Lithography
Semiconductor laser-interference lithography efficiently and quickly creates nanopatterns through two-laser beam interference on photoresist surfaces. These patterns are transferred to other materials using liftoff or chemical etching techniques.
The resulting nanostructures can be used in various applications such as high-density data storage, field emission flat-panel displays, and micron-scale perforated membranes.
Biological Applications
Semiconductor lasers can transmit optical energy to increasingly smaller modes with simultaneous temporal and spectral optical localization. This makes them useful for various biological applications, such as biological probing, super-resolution imaging, and cellular labeling.
Quantum Nanophotonics
Semiconductor lasers have found key applications in quantum nanophotonics. For example, they can produce single photons with high indistinguishability on demand, essential for photonic quantum computing and long-distance quantum communication.
Monolithically integrated semiconductor lasers provide a compact and scalable solution for producing photonic state generators and modular quantum light sources.
Nanolasers: Miniaturization of Semiconductor Lasers
Semiconductor lasers have undergone significant advancement with the emergence of nanoscience and nanotechnology. As a result, researchers have directed considerable efforts towards producing ultra-compact lasers with low lasing thresholds, leading to the development of sub-100 nm semiconductor lasers.
Some examples of these lasers are microcavity lasers, vertical-cavity surface-emitting lasers, polariton lasers, semiconductor wire lasers, photonic crystal lasers, and plasmonic nanolasers. These nanolasers have highly desirable and unique properties such as ultralow threshold, subwavelength, ultrafast laser operation, and diffraction-unlimited.
As a result, they have become a popular research field for various applications, such as low energy-consumption high-speed displays, biomedical imaging, ultrahigh-density data storage, and molecular communication devices.
Smallest Semiconductor Laser
In a study published in ACS Nano, international researchers developed the world’s smallest semiconductor laser. They created a 310-nanometer cube that, when photoexcited by an ultrashort laser pulse, emits laser at ambient temperature.
A conventional laser comprises two essential components: an optical resonator that aids in the long-term confinement of electromagnetic energy and an active medium that enables the development of coherent stimulated emission. In this study, researchers chose halide perovskite as it could simultaneously function as an efficient resonator and active medium.
The newly developed nanolaser has unique characteristics that extend beyond its small size. Its design enables efficient confinement of stimulated emission energy, producing high electromagnetic field amplification for laser generation.
The nanolaser can operate at atmospheric pressure and room temperatures without requiring external pressure or extremely low temperatures, making the technology appealing to sensors, optical chips, and other devices that process and transfer information using light.
Future Outlooks
The increasing trend of miniaturization in nanotechnology and electronics necessitates the creation of more efficient and compact light sources, making semiconductor lasers an attractive option.
Advances in nanotechnology have led to the development of new types of semiconductor lasers with superior properties, such as nanowire lasers, quantum dot lasers, and plasmonic lasers, which will lead to new and exciting applications in the future.
References and Further Reading
Deng, H., Lippi, G. L., Mørk, J., Wiersig, J., & Reitzenstein, S. (2021). Physics and Applications of High‐β Micro‐and Nanolasers. Advanced Optical Materials, 9(19), p. 2100415. https://doi.org/10.1002/adom.202100415
Hofmann, M. R., & Koch, S. W. (2022). Semiconductor Lasers. In Springer Handbook of Semiconductor Devices. Cham: Springer International Publishing. pp. 851-864. https://doi.org/10.1016/B978-0-12-803581-8.10103-1
Ma, R. M., & Oulton, R. F. (2019). Applications of nanolasers. Nature nanotechnology, 14(1), pp. 12-22. https://doi.org/10.1038/s41565-018-0320-y
Narlikar, A. V., & Fu, Y. Y. (Eds.). (2010). Laser Applications in Nanotechnology. Oxford Handbook of Nanoscience and Technology: Volume 3: Applications. OUP Oxford. doi.org/10.1093/oxfordhb/9780199533060.013.24
Tiguntseva, E., Koshelev, K., Furasova, A., Tonkaev, P., Mikhailovskii, V., Ushakova, E. V., ... & Makarov, S. V. (2020). Room-temperature lasing from Mie-resonant nonplasmonic nanoparticles. ACS nano, 14(7), pp. 8149-8156. https://doi.org/10.1021/acsnano.0c01468
Zhang, Q., Shang, Q., Su, R., Do, T. T. H., & Xiong, Q. (2021). Halide perovskite semiconductor lasers: materials, cavity design, and low threshold. Nano Letters, 21(5), pp. 1903-1914. https://doi.org/10.1021/acs.nanolett.0c03593
Disclaimer: The views expressed here are those of the author expressed in their private capacity and do not necessarily represent the views of AZoM.com Limited T/A AZoNetwork the owner and operator of this website. This disclaimer forms part of the Terms and conditions of use of this website.