The behavior of fluids confined within nanometer-thick layers at solid interfaces has received considerable attention in recent years due to its importance in biology, medicine, and material science.
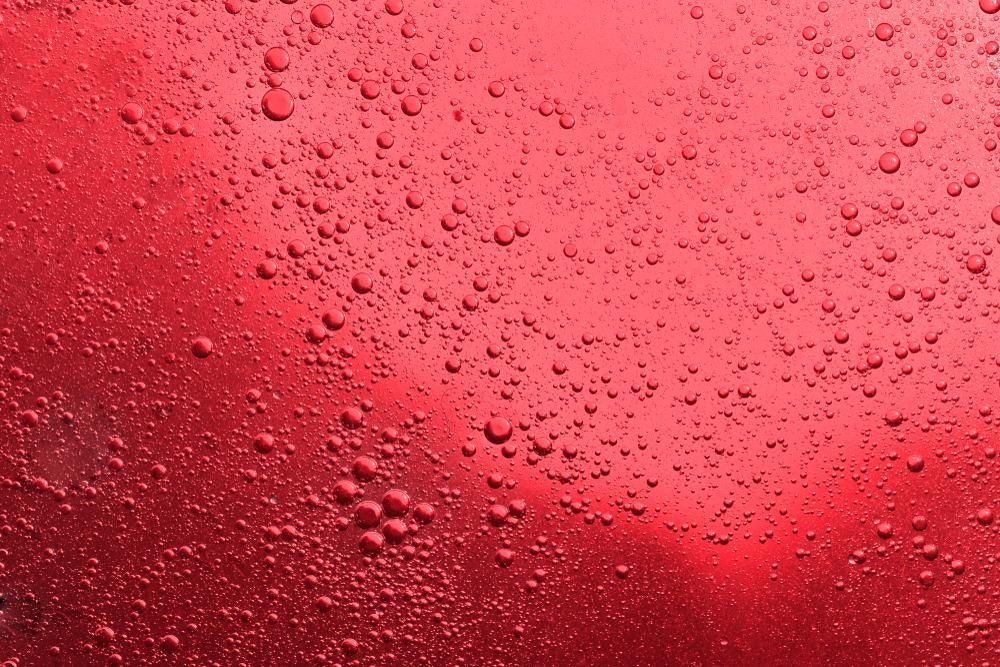
Image Credit: Beautyimage/Shutterstock.com
Over the past two decades, numerous experimental and theoretical studies have demonstrated that the behavior of nano-confined complex fluids differs remarkably from that at the macroscale.
Several nano-rheological techniques have been developed to investigate the phenomena that occur in such confined fluids.
Fluids flow when subjected to stress. There are many different types of fluids, such as pure substances, mixtures, dispersions, and solutions. These can be divided into two categories - simple and structured fluids.
Rheology is a well-established research discipline that studies how such materials respond to input stress. A material with a uniform composition (a single phase), such as a solution or pure substance, is described as a simple fluid.
Materials that contain more than one phase, such as solid nanoparticles dispersed in a liquid, gas particles in foam, or a nano-droplet emulsion of immiscible liquids, are considered structured fluids.
The rheological behavior of such fluids becomes dominated by the interactions of the constituent phases at a molecular scale. Besides, when such complex fluids are confined in geometries where at least one dimension approaches the molecular size (coatings, lubrication layers, biological membranes, and others), their rheological behavior becomes very complex as the classical laws that describe flow under stress break down.
Investigating the Nanomechanics and Nanorheology of Complex Materials
Measuring the viscoelastic behavior of thin layers of liquids, polymers, colloids, foams, and other biological materials, such as cells or tissues, confined in the vicinity of an interface is an experimental challenge.
These soft materials are usually viscoelastic and display strain rate-dependent mechanical properties. In addition, many advanced nanomaterials and nanocomposites are inhomogeneous and anisotropic, exhibiting features on multiple length scales resulting in neither ideal solid- nor liquid-like rheological behavior.
Rheometry and surface force apparatus measurements are well-established techniques that can characterize the rheology, or the stress-strain relationship between flow and deformation, of macroscopic soft matter and fluids. However, nanoscale methods for probing the viscoelastic heterogeneities inherent to soft matter specimens often require more sophisticated instrumentation.
Over the past decade, several experimental techniques have been developed that can capture both static and dynamic nanorheological properties of confined fluids.
In 2019, researchers from the Ecole Normale Supérieure in Paris demonstrated a redesigned surface force apparatus capable of probing the mechanical impedance of confined fluid in two dimensions (normal and tangential to the confined fluid layer).
The instrument employs a macroscopic tuning fork as a displacement sensor, and the sample under characterization is confined between a millimeter-sized sphere and a flat substrate attached to a piezo scanner.
The researchers demonstrated the technique's capabilities by measuring the micrometer-range rheological response of a silicone oils sample and the surface forces arising from the molecular-level confinement of the fluid under investigation.
Atomic Force Microscopy Reveals Mechanical Properties at the Nanoscale
Another highly versatile experimental technique, atomic force microscopy (AFM), is commonly used to explore the structure of complex soft materials, such as polymers, biomolecules, and biological membranes.
The operating principle behind AFM, where a tiny sharp probe interacts with and deforms the sample, allows the physical properties of soft materials to be measured.
The high spatial resolution of the AFM technique allows sample characterization on the atomic scale, while the force resolution is of the order of picoNewtons. Thus, the mechanical properties of soft materials can be characterized on a nanometer scale.
Several research groups have successfully employed AFM to explore the nanomechanics and nanorheology of a wide range of materials, ranging from polymer hydrogels, which are used as drug carriers, artificial tissue scaffolds, and in various biomedical applications devices, to rubber nanocomposites and live biological cells.
In addition, AFM-based nanorheology measurements enabled researchers to disentangle the physical processes behind an everyday-life phenomenon such as the slipperiness of ice, which remains controversial despite a long history of scientific research.
The in-situ investigation of the mechanical properties of the interfacial film of meltwater and its thickness under various load regimes and temperatures revealed complex viscoelastic rheology of the nanometer-thick water layer with a viscosity up to 2 orders of magnitude larger than bulk water.
These insights into the unconventional rheology of meltwater provided a new understanding of ice slipperiness and open possibilities for hydrophobic coatings that can reduce friction.
Nanorheology of Living Cells Measured by Gold Nanorod Tracking
A clear understanding of the nanorheology and friction at interfaces is of great importance for many biological and medical applications, such as lubrication and coating in joints and drug permeability in biological membranes.
Extensive research has demonstrated that the rheological properties of human blood can be used as a marker for the onset of cardiovascular disease.
A research group from the University of Pennsylvania in the USA has been able to study the nanorheology of lipid bilayer membranes in unprecedented detail by measuring the time-varying orientation of gold nanorods attached to the lipid bilayer, using high-speed dark-field optical microscopy.
The nanorod tracking approach proved to be a reliable method for measuring membrane properties and dynamics of the plasma membrane of single living cells.
Since nanorod orientational tracking is a polarimetric technique, the researchers envisage that by using faster wide-field cameras and brighter laser illumination, the method can simultaneously enable high-throughput measurements of different cells or cell regions.
Studying whole cells with rheology-based methodologies will be enabled, along with subcellular organelles or the dynamics of cell-cell interactions.
Continue reading: Why is Understanding the Rheology of Nanofluids Important?
References and Further Reading
Molaei, M., et al. (2021) Probing lipid membrane bending mechanics using gold nanorod tracking. arXiv:2104.12272v1. Available at: https://doi.org/10.48550/arXiv.2104.12272
Garcia, P. D., et al. (2020) Nanorheology of living cells measured by AFM-based force–distance curves. Nanoscale, 12, 9133-9143. Available at: https://doi.org/10.1039/C9NR10316C
Canale, L., et al. (2019) Nanorheology of Interfacial Water during Ice Gliding. Phys. Rev. X, 9, 041025. Available at: https://doi.org/10.1103/PhysRevX.9.041025
Beuguel, Q., et al. (2019) Nanorheology with a Conventional Rheometer: Probing the Interfacial Properties in Compatibilized Multinanolayer Polymer Films. ACS Macro Letters, 8 (10), 1309-1315. Available at: https://doi.org/10.1021/acsmacrolett.9b00662
Lainé, A., et al. (2019) MicroMegascope based dynamic surface force apparatus. Nanotechnology, 30, 195502. Available at: http://dx.doi.org/10.1088/1361-6528/ab02ba
Li, T.-D., et al. (2014) Nanorheology by atomic force microscopy", Review of Scientific Instruments, 85, 123707. Available at: https://doi.org/10.1063/1.4903353
Disclaimer: The views expressed here are those of the author expressed in their private capacity and do not necessarily represent the views of AZoM.com Limited T/A AZoNetwork the owner and operator of this website. This disclaimer forms part of the Terms and conditions of use of this website.