Approximately 90% of the atmospheric NH3 originates from agriculture, i.e., during livestock feeding and housing, fertilizer production, and manure storage. Many types of NH3 sensors have been developed to monitor and detect NH3. This article focuses on how nanomaterials are used to detect NH3 in agriculture.
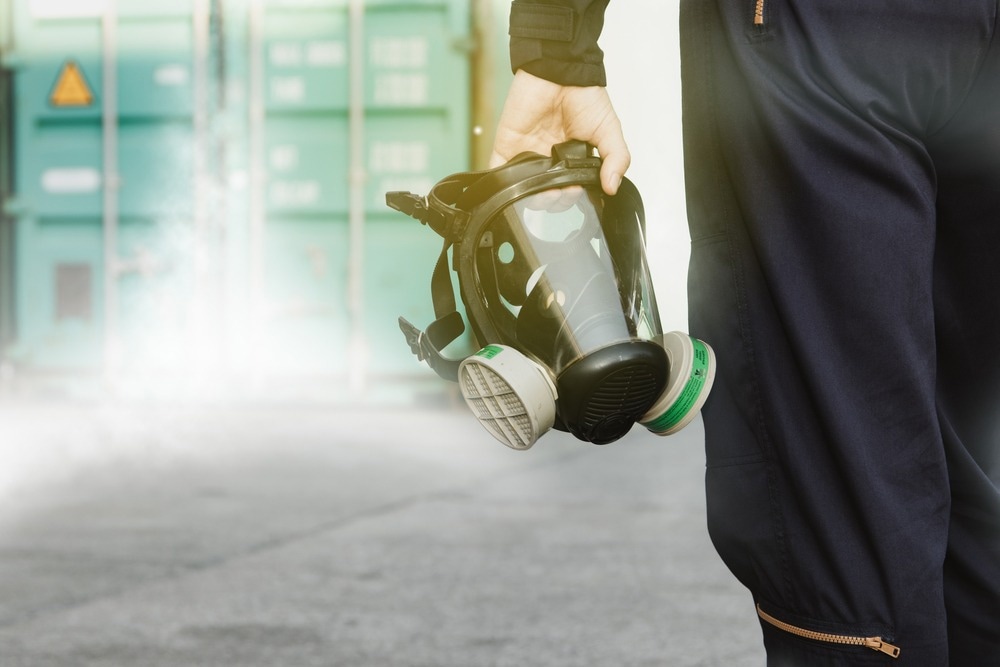
Image Credit: chalermphon_tiam/Shutterstock.com
Ammonia - A Toxic Gas
Ammonia is an alkaline compound that, when released into the air, is quickly adsorbed to surfaces and can travel anywhere ranging from a few hundred meters to hundreds of miles. NH3 is also converted into aerosol particles and has a distinct smell, which is commonly noticed in rural settings where a high density of poultry, pig, or cattle facilities prevail.
NH3 is a colorless gas with a distinct odor, even at low concentrations. This gas is commonly released after the decomposition of organic materials and animal waste. In the agriculture industry, NH3 is used to manufacture nitrogen-based fertilizers, such as ammonium nitrate, urea, ammonium sulfate, urea-ammonium nitrate, and diammonium phosphate.
The United States Environmental Protection Agency has regarded NH3 as a hazardous substance because it is toxic to humans. The maximum long-term (8 hours) and short-term (15 min) exposure to ammonia that has been considered to be safe for humans are 25 ppm and 35 ppm, respectively.
The Need for Ammonia Gas Detection in Agriculture
To develop a better agricultural system, it is important to identify the sources that contribute to NH3 emission, particularly the time and level of the emission. Most NH3 sensors are costly and difficult to apply in real settings. The complex operation of these devices imposes additional difficulty for non-specialist practitioners.
The complexity of the agricultural environment arising from varying humidity, temperatures, and interfering gases demands a stable, sensitive, and reliable gas sensor. Typically, NH3 sensors are based on polymers, metal oxide semiconductors (MOS), and nanomaterials and their composites. Although many NH3 sensors exhibit high sensitivity and accuracy in laboratory settings, they fail to perform well in actual field conditions.
Nanomaterials Improve Ammonia Sensing Properties in Sensors
Graphene, single-walled carbon nanotubes (SWCNTs), multi-walled carbon nanotubes (MWCNTs), graphene nanoribbons, and reduced graphene oxide have been used to improve the gas-sensing properties of NH3 sensors based on polyaniline (PANI). Incorporation of SWCNTs into PANI aids in the formation of a structure due to hydrogen bonds between PANI and carboxylated carbon nanotubes. This enhances the interaction between NH3 and SWCNTs–OH/PANI composite. SWCNTs functionalized with carboxylic groups are used in ammonia detection.
Although MWCNTs have been rarely used to detect NH3, nanocomposites comprising MWCNTs, polyaniline, and metal oxides (e.g., aluminum oxide and titanium oxide) were found to significantly improve the sensing behavior toward ammonia. Besides carbon nanotubes, carbon nanofibers (CNFs) have also been used to detect NH3. Notably, the porosity of CNFs is significantly higher than MWCNTs, which elevates their sensing characteristics. A meta-toluic acid-functionalized graphene oxide on a silicon dioxide/silica substrate, using Langmuir–Blodgett method, can detect ammonia in an agricultural field.
Most sensors in real settings, such as vegetable fields, fail to perform due to the presence of oxidative interference gases (O3 and NO2) in the surrounding air and elevated humidity levels. These shortcomings were overcome with an indirect approach, i.e., the catalytic conversion method (CCM). Here, NH3 was converted to nitrogen dioxide (NO2) using a platinum (Pt) nanoparticle catalyst, and this gas was detected by high-performance NO2 sensors.
A portable, low-cost, stable, and sensitive NH3 monitor has been recently developed based on a differential catalytic conversion method (DCCM). This device can effectively monitor ammonia volatilization in the agricultural sector and eliminates environmental interference. The key sensing material of this sensor is indium (III) oxide nanoparticles and tungsten oxide (WO3) nanosheets.
Different Types of Nanomaterial-based Sensors Used to Detect Ammonia Gas
The different types of nanomaterial-based sensors used to detect ammonia gas are discussed below:
Electrochemical Sensors
Electrochemical sensors detect changes in electrical signals caused due to adsorption of reduction into different materials. In agriculture, chemo-resistive materials are commonly used to detect reducing or oxidizing gases, based on the charge transfer between the sensing element and the redox gas.
Many times, these detectors are developed using metal nanomaterials and conductive polymers. The application of polyaniline nanocomposites, i.e., polyaniline coupled with carbon nanotubes, graphene, and other carbon nanomaterials, has resulted in the production of stable, sensitive, and reliable NH3-sensing material.
Spectrometry-based Sensors
Spectrometry-based sensors are highly stable, have strong resistance to electromagnetic noise, and consume low energy for their operation. Absorbance-based and colorimetric sensors are commonly available, which are used to measure NH3-derived compounds. Absorbance-based sensors are best for continual monitoring over a large area.
For spectrometric analysis, tubes are available with different measurement ranges. Even though a range between 0.25 and 30 ppm is suitable for indoor detection of NH3, it is not adequate for outdoor analysis. To overcome this problem, nano-based thin-film samplers have been developed. For instance, a thin dye-doped nanostructure polypyrrole film has been developed, which indicates the presence of NH3 in optical absorption.
Fluorescence-based Sensors
Fluorescence is not commonly used to measure air quality in agriculture settings because very few relevant compounds fluoresce. For fluorescence-based sensing, recently, there has been a growing interest in replacing conventional organic dyes with carbon-based photo-luminescent nanomaterials.
The main advantages of this type of sensor in agricultural sectors are high sensitivity, lower cost, higher portability, and easier design. It has been observed that photo-luminescent carbon dots (CDs) are superior to previously used semiconductor nanomaterials, in terms of their low toxicity, affordability, and high biocompatibility. Fluorescent CDs have been able to determine NH3 from both solid and liquid agricultural samples. In this context, simultaneous extraction and fluorimetric labeling of NH3 takes place, followed by fluorescence measurement.
Since the NH3 molecule is not fluorescent by nature, quantifications are done based on derivatization reactions. A porous matrix or trapping solution produces a fluorescent signal from the NH3 molecule.
References and Future Reading
Jian, A. et al. (2022) Synthesis of MoS2 Nanochains by Electrospinning for Ammonia Detection at Room Temperature. ACS Omega. 7(14), pp.11664-11670. https://pubs.acs.org/doi/10.1021/acsomega.1c06456.
Yang, B. et al. (2022) A low cost and high performance NH3 detection system for a harsh agricultural environment. Sensors and Actuators B: Chemical. 361, p. 131675. https://www.sciencedirect.com/science/article/abs/pii/S0925400522003173?via%3Dihub
Chugh, B. et al. (2022) Nanoparticles-based sensors for agricultural application. Nanosensors for Smart Agriculture. 706(4). https://www.sciencedirect.com/science/article/abs/pii/B9780128245545000094?via%3Dihub
Bannov, A.G. et al. (2021) Recent Advances in Ammonia Gas Sensors Based on Carbon Nanomaterials. Micromachines. 12(2), p. 186. https://www.mdpi.com/2072-666X/12/2/186
Wang, Y. et al. (2021) Conductometric room temperature ammonia sensors based on titanium dioxide nanoparticles decorated thin black phosphorus nanosheets. Sensors and Actuators B: Chemical. 349. https://www.sciencedirect.com/science/article/abs/pii/S0925400521013381?via%3Dihub
Disclaimer: The views expressed here are those of the author expressed in their private capacity and do not necessarily represent the views of AZoM.com Limited T/A AZoNetwork the owner and operator of this website. This disclaimer forms part of the Terms and conditions of use of this website.