Polymeric nanoparticles are nanoscale-sized objects constructed from polymers such as polyethylene glycol (PEG) or polylactide-co-glycolide (PLGA), with applications in the in vitro delivery of therapeutic and diagnostic agents, alongside a number of additional biomedical functions. In this article, AZoNano covers the main properties and applications of polymeric nanoparticles.
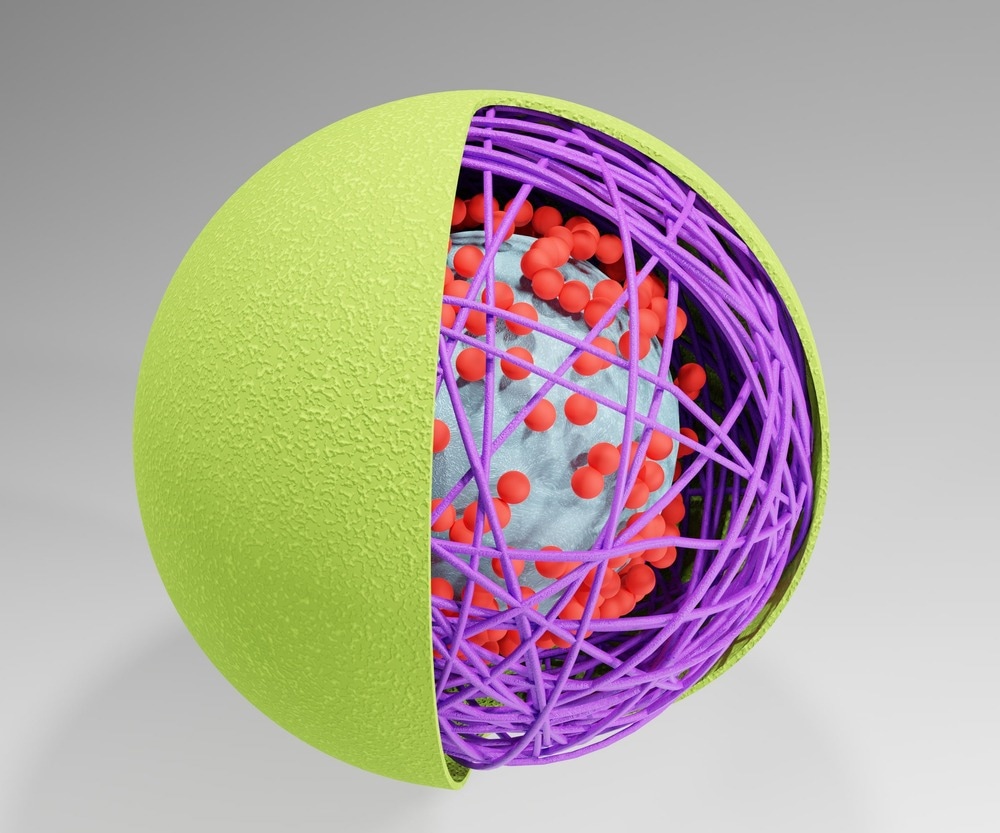
Image Credit: Love Employee/Shutterstock.com
When formed into a nanoparticle, any polymer, that is, a molecule constructed of monomers, could be considered a polymeric nanoparticle, including liposomes constructed from cholesterol monomers, and even extremely long single-chain polymers wrapped and tangled upon themselves to produce a single spherical polymeric nanoparticle.
Polymeric Nanoparticles: A History
Polymeric nanoparticles intended for applications in medicine have been under exploration for several decades, with liposomes and micelles constructed from amphipathic lipid molecules being loaded with drugs as early as the 1970s in the lab.
The advantages associated with polymeric nanoparticles, such as enhanced cargo solubility and improved in vivo retention time, were recognized early, and a variety of drug and diagnostic cargo was investigated for improved drug delivery systems.
By the late 1970s and 1980s, polymeric nanoparticles had been constructed from a variety of other monomers, and differences in biodistribution, elimination, and other biointeractions were noted dependent on particle surface chemistry and morphology.
During the 1990s, massive advancements were made in polymeric nanoparticle biocompatibility by incorporating inert coatings made from PEG, wherein the particle is imbued with “stealth” properties that allow it to avoid early excretion by circulating phagocytes.
Protein and nucleic acid therapeutics became a realistic possible treatment option in the 2000s, and their delivery by polymeric nanoparticles was investigated early. Biomolecules such as these are quickly degraded by a variety of mechanisms once administered and thus require protection until they are released at the target site.
More recently, these technologies were combined in the massively successful COVID-19 vaccines, wherein sensitive mRNA was delivered by liposomal polymeric nanoparticles.
What Are Polymeric Nanoparticles?
Polymeric nanoparticles share many of the advantages associated with nanomedicines in general, namely enhanced bioavailability of otherwise poorly absorbed drugs, usually associated with poor water solubility, but also potentially owing to poor bioretention and quick elimination, as in the case of sensitive therapeutic proteins or nucleic acids.
Like other nanoparticles, polymeric nanoparticles possess a large surface area, allowing a large quantity of drug cargo and other functional or protective small molecule agents to be bound to the surface.
Unlike nanoparticles constructed of dense solid materials such as gold, polymeric nanoparticles have much of their internal volume available for the carriage of drug cargo, either within a hollow polymer shell or mixed in with a porous low-density polymer structure throughout the particle. This allows the exterior surface of the polymeric nanoparticle to be dedicated to protective and targeting functions while a massive payload of drug cargo can be simultaneously delivered to cells from within the internal volume of the particle.
Polymers such as PEG are already commonly utilized as biocompatible coatings for nanoparticles constructed from other materials; thus, polymeric nanoparticles avoid early elimination by circulating phagocytotic cells while being easily degraded once within the lysosomal compartments of target cells.
Unfortunately, many of the innate advantages of metal nanoparticles, such as high z-number and unique optical properties, are lacking from polymeric nanoparticles. However, by avoiding concerns associated with bioaccumulation and toxicity, polymeric nanoparticles may have an important role to play in targeted drug delivery.
How Are Polymeric Nanoparticles Made?
Polymeric nanoparticles can be produced from either top-down or bottom-up methods, that is, by breaking down bulk polymer materials or by growing from the polymerization of monomers. Drug or other sensitive cargo can be encapsulated within polymeric nanoparticles as they form or bound to the surface later using a wide variety of chemical linkers.
One of the simplest methods of generating polymeric nanoparticles with encapsulated cargo is emulsification, in which an organic solution containing the monomer and cargo is dripped into an aqueous solution.
Spherical droplets of organic fluid remain suspended, allowing the hydrophilic end of the monomer to self-assemble towards the aqueous exterior in a manner similar to lipids, simultaneously entrapping the cargo inside the polymeric nanoparticle.
Excess water can then be removed by evaporation or ‘salting out’ by osmosis, which encourages polymerization. The ratio between polymer and oil content can vary greatly between polymeric nanoparticles, becoming a completely solid polymer or retaining a liquid core surrounded by an approximately 5 nm thick polymer envelope.
These polymeric nanoparticles tend to be in the order of one to a few hundred nanometers, though smaller particles can be generated by micro-injection of organic polymer and drug solution.
Where Are Polymeric Nanoparticles Used?
Polymeric nanoparticles are primarily of interest to the biomedical and biochemical fields, where their biocompatibility, nanoscale characteristics, and large and customizable surface and interior volume can be exploited for a variety of purposes.
In the lab, the ability to bind numerous smaller biochemical indicators to a single platform with greater mass is highly advantageous. For example, multiple green fluorescent proteins can be bound to a single polymeric nanoparticle, intensifying and localizing the fluorescent signal when they are all simultaneously activated.
Polymeric nanoparticles with this design can be exploited in the clinic to develop biosensors with high specificity towards the target site when binding both targeting and sensing ligands to the particle, alongside other drug delivery purposes.
Polymeric nanoparticles and nanocomposites are also explored as antimicrobial food packaging materials with antioxidant properties, offering numerous specific advantages over traditional polymer materials.
Nanostructured polymers constructed using polymeric nanoparticles offer improved physical properties such as flexibility, temperature and moisture sensitivity, durability, and protection from water or gas ingress.
Some specific types of polymeric nanoparticles, such as those made from chitosan, are known to possess antimicrobial properties, or alternatively, other antimicrobial materials, such as silver nanoparticles, can be incorporated into the polymeric nanoparticle food packaging material.
Nanoparticle sensors can also be incorporated into “smart packaging” materials as described above, by incorporating colorimetric or fluorimetric molecules or specific polymeric nanoparticles into the material that alters its optical properties in the presence of, for example, moisture, extreme temperatures, a break in packing integrity, or the presence of pathogens.
Polymeric nanoparticle composite materials such as these can be produced by a number of methods, such as layer-by-layer deposition, emulsification of monomers directly into shape, and electrospinning from nanofibers.
References and Further Reading
Vauthier, C. (2019). A journey through the emergence of nanomedicines with poly (alkylcyanoacrylate) based nanoparticles. HAL Open Science. Available at: https://hal.science/hal-02359395/document
Zielińska, A., Carreiró, F., Oliveira, A. M., Neves, A., Pires, B., Venkatesh, D. N., Durazzo, A., Lucarini, M., Eder, P., Silva, A. M., Santini, A., & Souto, E. B. (2020). Polymeric Nanoparticles: Production, Characterization, Toxicology and Ecotoxicology. Molecules, 25(16), p. 3731. doi.org/10.3390/molecules25163731
Vasile, C.. (2018). Polymeric Nanocomposites and Nanocoatings for Food Packaging: A Review. Materials, 11(10), p. 1834. doi.org/10.3390/ma11101834
Disclaimer: The views expressed here are those of the author expressed in their private capacity and do not necessarily represent the views of AZoM.com Limited T/A AZoNetwork the owner and operator of this website. This disclaimer forms part of the Terms and conditions of use of this website.