A recent study available as a pre-proof in the journal Carbon examines the plasmonic effects of silver and gold nanoparticles of different geometries to regulate and improve the fluorescence intensity of Single-Walled Carbon Nanotubes (SWCNTs).
Study: Plasmon-induced near-infrared fluorescence enhancement of single-walled carbon nanotubes. Image Credit: Oselote/Shutterstock.com
It was observed that gold (Au) nanorods increase chirality emissions by 80 % whereas silver (Ag) nano triangles increase emissions by 200 %. Finite element modeling (FEM) was used to demonstrate the plasmon-induced localized increase in electron density as well as radiative recombination of dark excited states from the resultant electromagnetic field.
What are Plasmonic Nanoparticles?
Plasmonic nanomaterials are metallic nanoparticles with diameters below the incoming light's wavelength. These nanostructures have enhanced light absorption and scattering capabilities at the resonance frequency.
Optical characteristics vary with particle size, shape, and chemical composition spanning wavelengths from ultraviolet (UV) to near-infrared (NIR-II) radiations. Infrared absorption, surface-enhanced Raman scattering (SERS), and energy harvesting are examples of applications based on plasmonic nanoparticles' optical tunability.
Plasmonic nanoparticles have been employed extensively in the past decade to improve the fluorescence characteristics of optically connected fluorophores. The plasmonic nanostructure's resonant wavelength matches the optical characteristics of the fluorescent dyes. Several studies have shown enhancement for a variety of fluorophores. In particular, the NIR-II range is employed for in vivo optical imaging applications.
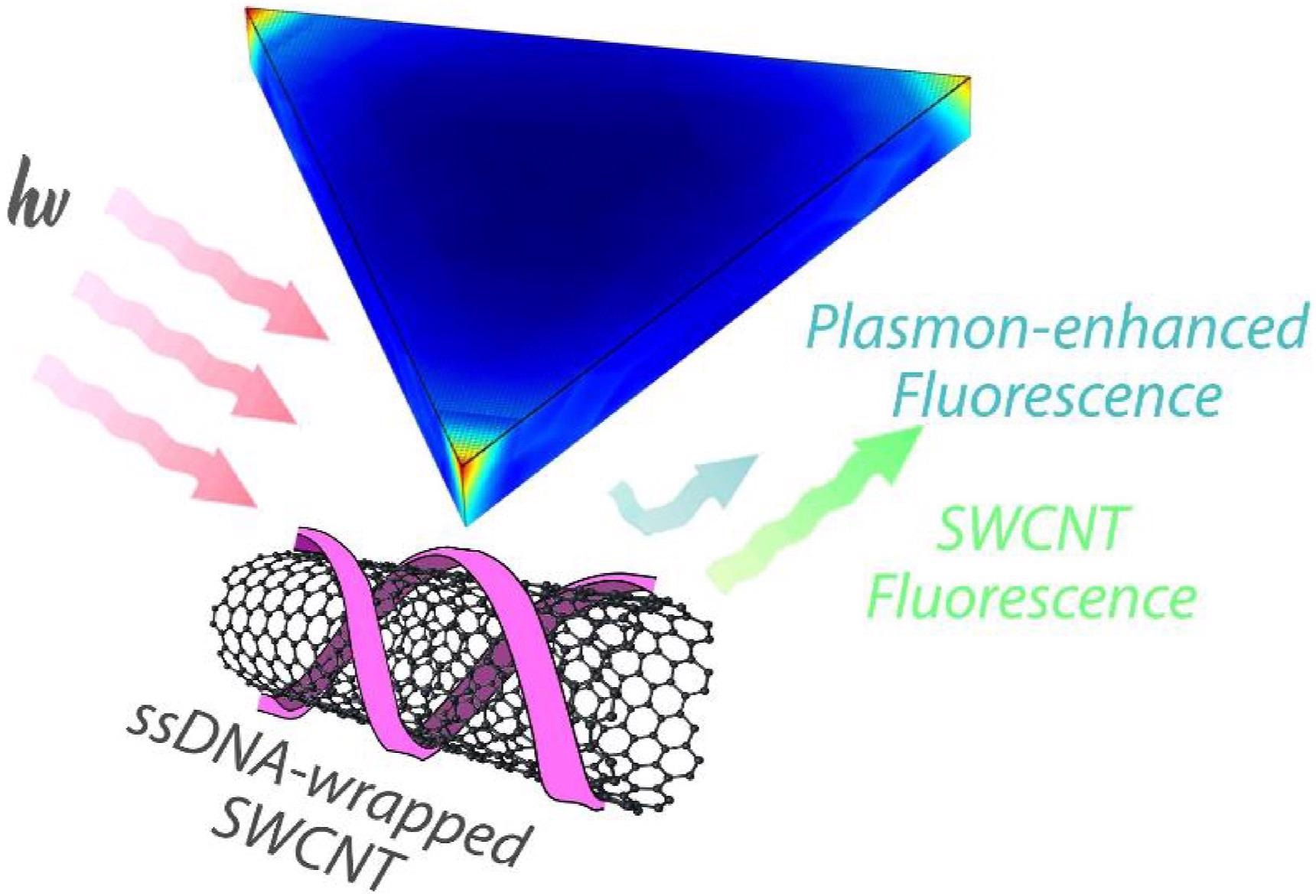
Figure 1. Schematic of ssDNA-wrapped SWCNT in the vicinity of metallic nanostructures that contribute to plasmon-enhanced fluorescence. Upon illumination at resonant wavelengths (red), the metallic nanoparticles show shape, size, and composition-specific plasmonic effects (blue) for enhancing NIR-II SWCNT fluorescence (green). © Amirjani, A. et al. (2022).
Characteristics of SWCNTs
SWCNTs have unique NIR-II fluorescence for in vivo imaging and sensing applications. The absorption and emission peaks change with nanotube diameter according to the indices of chirality.
SWCNTs emit NIR-II inside the optical transparency window of biological materials, where biofluids and tissues are little absorbed. In addition to the large penetration depths at longer wavelengths, these emissions have unlimited photostability, making them ideal for continuous deep tissue imaging in biological samples.
SWCNTs exhibit modest fluorescence quantum yields (QYs), ranging from 0.1 to 1.5 percent in aqueous environments. The increased sensitivity of optical sensors, which use brighter nanotubes, might be attributed to increased QYs.
Due to the absence of scalable, repeatable, and accessible SWCNT synthesis processes, long, defect-free SWCNTs are difficult to fabricate. Therefore, metal-enhanced fluorescence of SWCNTs mounted on substrate surfaces has been used to increase QY. Despite the 10-fold increase in fluorescence, the SWCNTs' surface immobilization restricts their application for solution-phase imaging. Adding reductive brighteners like Trolox may improve QY, but at the price of cytotoxicity and sensor sensitivity.
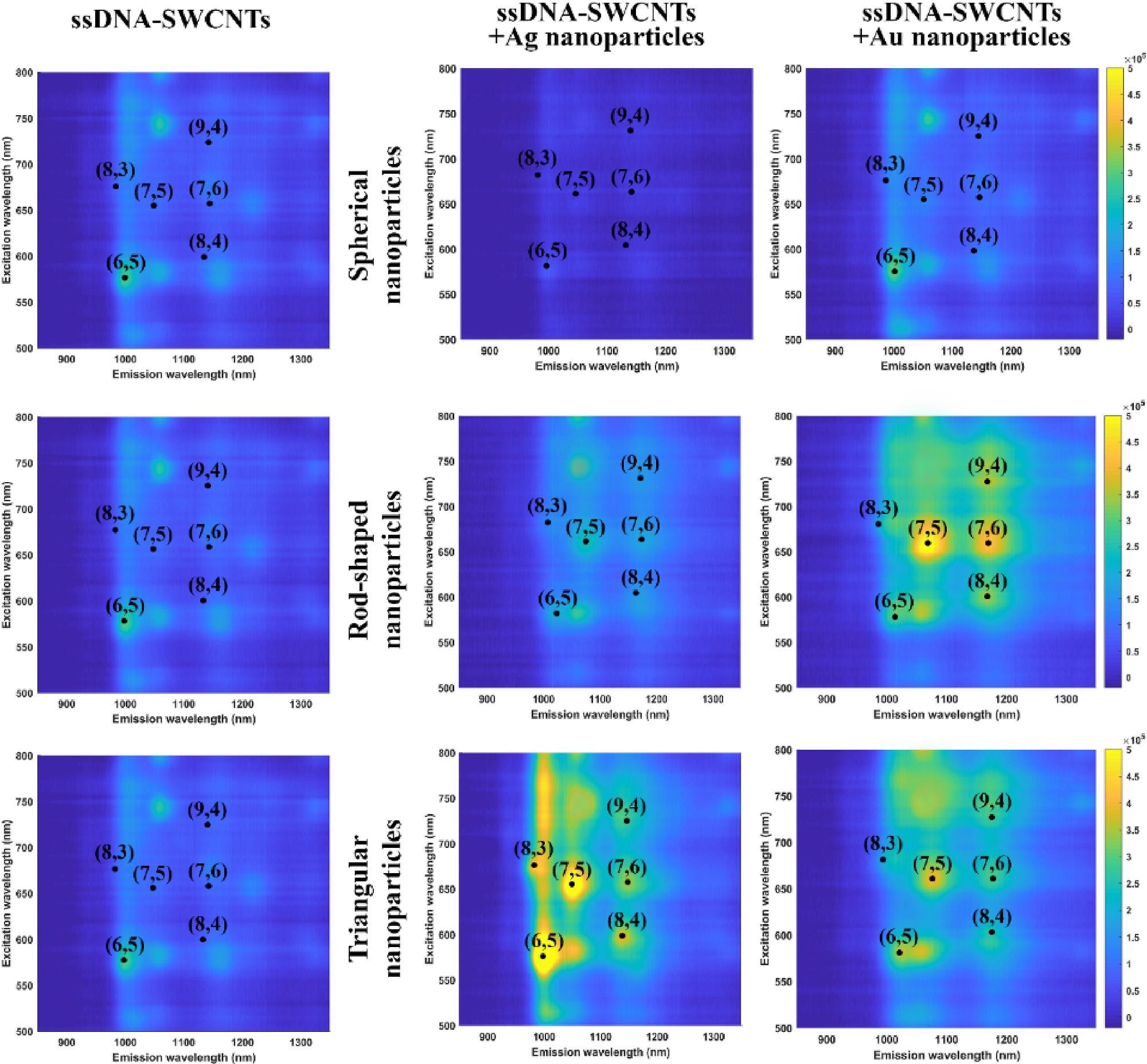
Figure 2. Excitation-emission maps for ssDNA-SWCNTs conjugated with spherical (top row), rod (middle row), and triangular (bottom row) nanoparticles. Maps are compared between pristine ssDNA-SWCNTs (left column), as well as ssDNA-SWCNTs in the presence of Ag nanoparticles (middle column) and Au nanoparticles (right column). © Amirjani, A. et al. (2022).
Plasmonic Interaction: A Novel Way to Improve SWCNT Characteristics
Plasmonic interaction with metal nanoparticles is a feasible way to improve SWCNT optical characteristics. Plasmonic nanoparticles have been shown to improve SWCNT Raman scattering for SERS imaging and photothermal treatment.
Several studies have employed plasmonic nanoparticles to boost near-infrared fluorescence of different fluorophores, but few have shown NIR-II SWCNT enhancement. A previous research work found a 20-fold increase in the impacts of gold nanorods. Despite the tremendous improvements, surfactant-suspended SWCNTs have limited optical sensing applications.
No other nanoparticle shapes, especially those with improved localized fields, have been examined previously and there is currently no systematic investigation assessing the influence of geometry, composition, and other parameters on adjusting plasmonic effects. SWCNTs have yet to be used to study the impacts of triangular Ag nanoparticles, which have greater plasmonic characteristics.
Detailed comparative research is therefore critical for understanding, modeling, and forecasting SWCNT plasmonic effects. In this study, the researchers investigated the impacts of spherical, rod, and triangle Ag and Au plasmonic nanostructures. Finite element modeling (FEM) was used to predict the impacts of nanoparticle shape and material, including near-field effects.
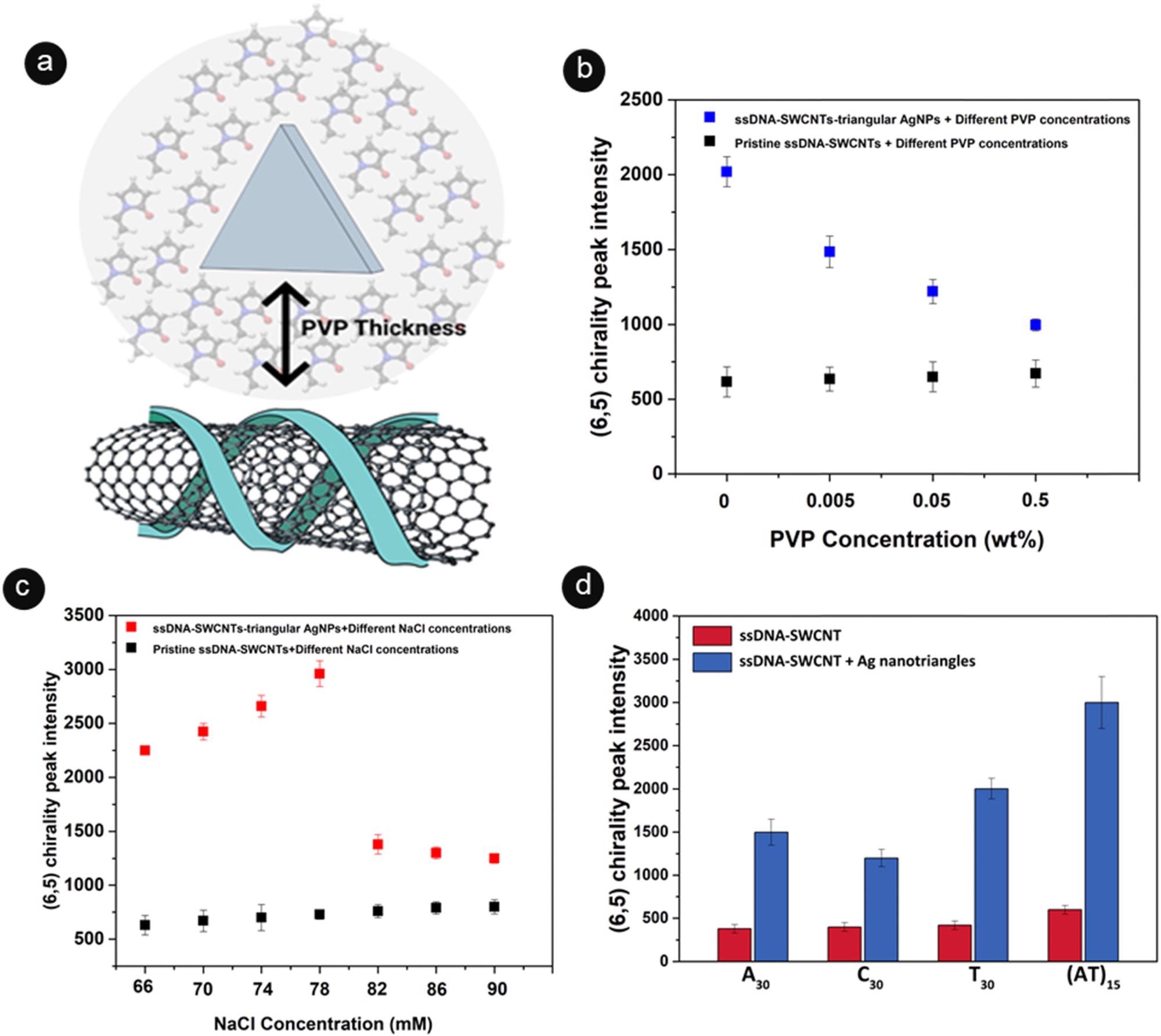
Figure 3. The effect of nanoparticle distance, salt concentration, and DNA sequence on the photoluminescence of ssDNA-SWCNTs. (a) Schematic representation of the effect of PVP thickness on modulating distance between plasmonic nanoparticles and ssDNA-SWCNTs. (b) Fluorescence intensity of (Eq. 6), (Eq. 5) ssDNA-SWCNT emission peak in the presence of Ag nanotriangles and varying PVP concentrations (0 - 0. 5 wt % PVP). Control measurements are shown for ssDNA-SWCNTs in the absence of the nanoparticles. (c) Fluorescence intensity of (Eq. 6), (Eq. 5) ssDNA-SWCNT emission peak in the presence of Ag nanotriangles and varying salt concentrations (66–90 mM NaCl). Control measurements are shown for ssDNA-SWCNTs in the absence of the nanoparticles. (d) Fluorescence intensity of (Eq. 6), (Eq. 5) ssDNA-SWCNTs suspended with AT15, A30, and T30 sequences in the absence (red) and the presence (blue) of Ag nanotriangles. © Amirjani, A. et al. (2022).
Research Conclusion
Here, it was shown that the plasmonic nanoparticles' fluorescence amplification is particularly useful for NIR sensing and imaging single-molecules. Confocal NIR imaging of SWCNTs was conducted as well, which has never been reported before. Using nanoparticle coupling, the constraints of poor quantum yields and large microscope fluorescence losses can be overcome.
Using confocal microscopy, the researchers found up to a 240 percent increase in fluorescence emissions and a single-molecule sensor's responsiveness. This is only one of several SWCNT applications that can benefit from the improved fluorescence shown in this study. Therefore, plasmonic nanoparticles can be used to enhance optical sensing and to modulate SWCNT fluorescence for useful changes in fundamental transitions of prohibited states.
Reference
Amirjani, A. et al. (2022). Plasmon-induced near-infrared fluorescence enhancement of single-walled carbon nanotubes. Carbon. Available at: https://www.sciencedirect.com/science/article/pii/S0008622322002123
Disclaimer: The views expressed here are those of the author expressed in their private capacity and do not necessarily represent the views of AZoM.com Limited T/A AZoNetwork the owner and operator of this website. This disclaimer forms part of the Terms and conditions of use of this website.