Magnetic particle imaging is a novel imaging technique that allows for quantitative direct imaging of superparamagnetic iron oxide nanoparticles (SPIONs). These advanced nanoparticles have shown great potential for applications in cancer diagnosis, enhanced targeting, cancer therapy and post-therapy monitoring, which will be further discussed in this article.
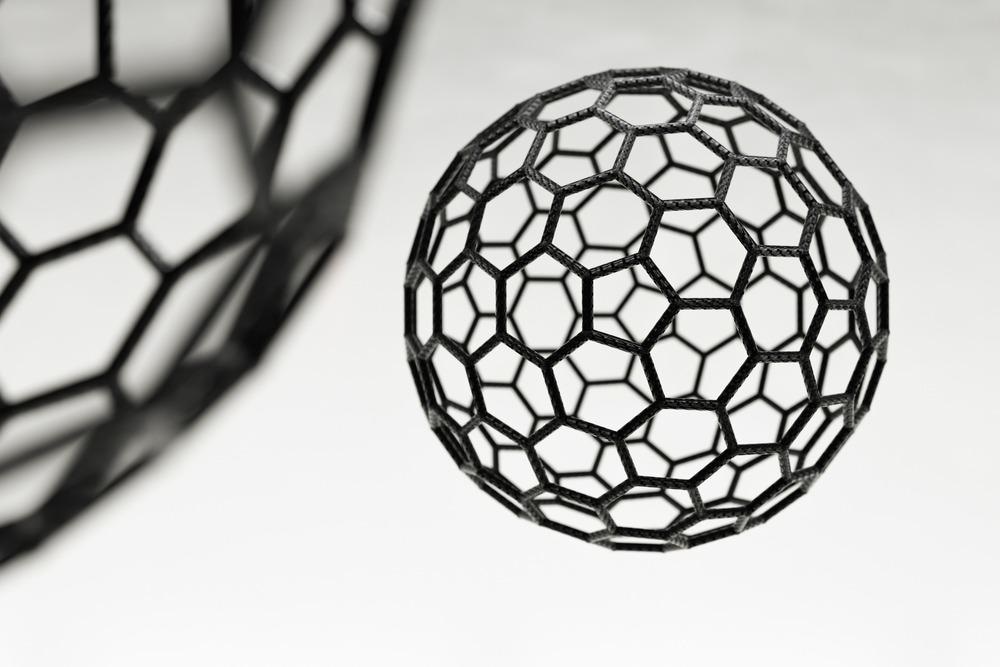
Image Credit: Forance/Shutterstock.com
Advancements in preventative medicine and therapeutics have illustrated a need for enhanced sensitivity and precision within both diagnostic and therapeutic procedures.
Magnetic particle imaging (MPI) was first proposed by Gleich and Weizenecker in 2005, which involves applying static and dynamic magnetic fields through the unique characteristics of superparamagnetic iron oxide nanoparticles.
It is a novel non-invasive technique that utilizes particles sized between 1 to 100 nanometers and has the potential to revolutionize biomedical research and medicine, from drug delivery systems to diagnostic imaging and cancer therapy.
Magnetic Particle Imaging vs. Magnetic Resonance Imaging
Magnetic particle imaging would be a welcome addition for use in clinical diagnostics and therapeutics along with other imaging techniques such as magnetic resonance imaging (MRI).
While this magnetic-based imaging modality is quite similar in name to MRI, the principles it operates on are drastically different. Magnetic resonance imaging utilizes a signal from the precession of nuclear spin magnetic moments of target nuclei; however, a magnetic particle imaging signal is typically from the ensemble magnetization of superparamagnetic iron oxide nanoparticles.
Magnetic particle imaging retains the benefit of zero tissue background signal that can occur in MRIs due to none of the iron oxide nanoparticles being present in native biological tissue, such as within patients. As a result, high image contrast can be achieved.
Results are comparable to tracer images found when using nuclear medicine scans, including positron emission tomography (PET) or single-photon emission computerized tomography (SPECT), which is currently the gold standard for diagnostic cancer imaging.
The difference is in the use of non-radioactive SPIONs with 20-100 nm in size.
Additionally, magnetic particle imaging works within the kilohertz frequency range, which enables magnetic fields to fully penetrate tissue, bone, and air without view limitations. It also produces effective results for lungs and bones, which are challenging for MRIs and ultrasounds.
Nanoparticles in Magnetic Particle Imaging
Superparamagnetic iron oxide nanoparticles can be synthesized through methods such as co-precipitation, thermal decomposition, hydrothermal synthesis, microemulsion, electrochemical synthesis, and bacterial synthesis.
Additionally, these nanomaterials are the most common type of magnetic nanoparticle used in magnetic particle imaging, and their magnetic characteristics can be seen when an external magnetic field is applied.
The controllable size, high surface area to volume ratio, as well as non-toxicity and surface functionalization of these magnetic iron oxide nanoparticles can be used to target desired sites through the application of an external magnetic field and allow direct and specific therapeutic effects with minimal adverse effects.
Research into these particles has illustrated their use in a wide range of applications other than medical imaging, such as surface functionalization with antimicrobial agents, which can be used to direct the particles to areas of infection, with direct delivery into the pathogen zone without affecting the whole organism. Additionally, superparamagnetic iron oxide nanoparticles have also been infused intravenously to detect lesions within the liver.
Applying an external magnetic field enables alignment with the magnetic moments of these iron oxide nanoparticles to produce a net magnetization vector that generates a signal for magnetic particle imaging. The magnetic behavior of SPIONs can be explained through the Langevin theory which assumes these nanoparticles are in a state of constant thermal equilibrium.
This theory is premised on the assumption of continual movement from SPIONs, which also possess randomly aligned magnetic moments resulting in zero net magnetization. However, when an external magnetic field is applied, these magnetic moments will align and cause a net magnetization vector.
Ultimately, the fixed magnetization response is a critical component for spatial encoding as well as the effective production of magnetic particle imaging results.
Translational Significance of Magnetic Particle Imaging
While the limitations of magnetic resonance imaging have been outlined, this conventional imaging technique is commonly utilized as a diagnostic tool for many disorders, from cancer to cardiovascular imaging. However, the reliance on MRI amongst others has illustrated issues such as long scan times, gadolinium exposure from MRI use, and radiation exposure from nuclear imaging procedures such as PET and CT scans.
The translation of magnetic particle imaging, while relevant for biomedical research and personalized medicine in general, has associated challenges. This includes the formulation of magnetic iron oxide nanoparticles, obtaining the appropriate level of signal sensitivity, and evaluating their chemical composition and toxicity in vivo.
Safety concerns can also include peripheral nerve stimulation and tissue heating, with the former a limiting factor for MRI and a potential risk factor for magnetic particle imaging use.
With MPI systems currently being available for rodent use, the upscale process of this scanner facilitating human imaging may also be a practical obstacle for this technique.
However, the advancement of nanotechnology and the use of nanoparticles in magnetic particle imaging have illustrated the value medical imaging technique can bring to conventional clinical diagnostics.
The necessity of a safe, ultra-sensitive and multipurpose medical imaging tool that can produce quantifiable 3D images, is a role that can be comfortably filled by magnetic particle imaging. As additional research is conducted, the translation of this technique into precision medicine is furthered.
References and Further Reading
Billings, C., Langley, M., Warrington, G., Mashali, F. and Johnson, J., (2021) Magnetic Particle Imaging: Current and Future Applications, Magnetic Nanoparticle Synthesis Methods and Safety Measures. International Journal of Molecular Sciences, 22(14), p.7651. Available at: https://doi.org/10.3390/ijms22147651
Han, X., Li, Y., Liu, W., Chen, X., Song, Z., Wang, X., Deng, Y., Tang, X. and Jiang, Z., (2020) The Applications of Magnetic Particle Imaging: From Cell to Body. Diagnostics, 10(10), p.800. Available at: https://doi.org/10.3390/diagnostics10100800
Menon, P., Sharma, A., Lafuente, J., Muresanu, D., Aguilar, Z., Wang, Y., Patnaik, R., Mössler, H. and Sharma, H., (2017) Intravenous Administration of Functionalized Magnetic Iron Oxide Nanoparticles Does Not Induce CNS Injury in the Rat: Influence of Spinal Cord Trauma and Cerebrolysin Treatment. International Review of Neurobiology, pp.47-63. Available at: https://doi.org/10.1016/bs.irn.2017.08.005
Panagiotopoulos, N., Vogt, F., Barkhausen, J., Buzug, T., Duschka, R., Lüdtke-Buzug, K., Ahlborg, M., Bringout, G., Debbeler, C., Gräser, M., Kaethner, C., Stelzner, J., Medimagh, H. and Haegele, J., (2015) Magnetic particle imaging: current developments and future directions. International Journal of Nanomedicine, p.3097. Available at: https://doi.org/10.2147/IJN.S70488
Seabra, A., Pelegrino, M. and Haddad, P., (2017) Antimicrobial Applications of Superparamagnetic Iron Oxide Nanoparticles. Nanostructures for Antimicrobial Therapy, pp.531-550. Available at: https://doi.org/10.1016/B978-0-323-46152-8.00024-X
Tay, Z., Chandrasekharan, P., Fellows, B., Arrizabalaga, I., Yu, E., Olivo, M. and Conolly, S., (2021) Magnetic Particle Imaging: An Emerging Modality with Prospects in Diagnosis, Targeting and Therapy of Cancer. Cancers, 13(21), p.5285. Available at: https://doi.org/10.3390/cancers13215285
Disclaimer: The views expressed here are those of the author expressed in their private capacity and do not necessarily represent the views of AZoM.com Limited T/A AZoNetwork the owner and operator of this website. This disclaimer forms part of the Terms and conditions of use of this website.