A variety of naturally occurring bioactive plants for the treatment of many diseases exist, and these plants have been widely used for millennia around the world due to their small side effects and significant health benefits.
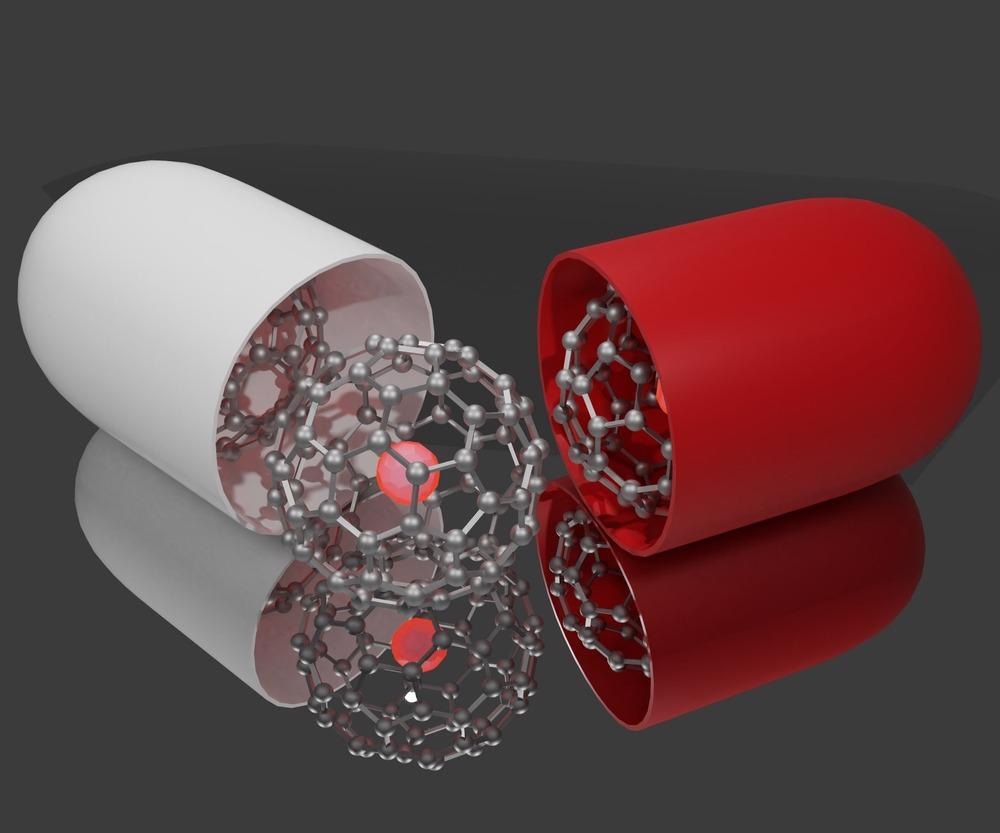
Image Credit: Love Employee/Shutterstock.com
Plants have been used for medicine and nourishment since the dawn of society. Despite the various benefits, pharmaceutical companies are often hesitant to fund natural product-based drug development and instead focus on the synthetic compound library for innovative drug development.
Natural products and phytoconstituents, on the other hand, have been extracted and screened for their advantages in basic health issues such as diabetes, cancer, microbial diseases, heart disease, and inflammatory conditions due to their unique benefits—which include reduced toxicity, low cost, fewer side effects, and superior health benefits.
Plant-based medicines have some drawbacks, such as low lipid solubility, instability, and the need for a well-validated method for ingredient extraction and purification. Furthermore, manufacturers must overcome these restrictions and ensure that the product is stable enough for patients to consume safely.
Newer improved drug delivery systems (DDS) for plant-based therapies have been designed to eliminate these constraints. Liposomes, phytosomes, ethosomes, transferosomes, nanostructured lipid carriers (NLCs), cubosomes, solid lipid nanoparticles (SLNs), hexosomes, microspheres, nanoparticles, and nanoemulsions are all examples of these.
Medicinal regulators have recently approved the first nanoparticle-based compositions containing lipid systems such as liposomes and micelles. Inorganic nanoparticles, such as magnetic and gold nanoparticles, were present in both formulations.
The physicochemical features of drug molecules determine the selection of nano-based formulations for drug administration at a specific place. Nanoscience has been increasingly popular in recent years for incorporating natural bioactives into nanoparticles.
The majority of the materials utilized are environmentally benign, biodegradable, bioadhesive, and of natural origin, providing a wide range of benefits as well as a unique size for the nano-formulations.
The biochemical and biophysical features of target drugs have largely determined the usage of a suitable medication and nano-DDS. Nonetheless, some drawbacks, such as toxicity, could not be overlooked in light of the advantages. The lack of knowledge about the toxicity and harmfulness of nanostructures is the primary source of concern, and more extensive investigations are unquestionably required to determine their maximal safety performance.
The current review paper published in the journal Pharmaceutics aims to describe natural products based on nano-DDSs, the widespread use of natural nanomedicines in various diseases, and various preparation techniques.
Discussion
Nanoscale materials have been created due to technological advancements over the last two decades, resulting in smaller particle sizes and larger surface areas. Nanoparticles are tiny particles that range in size from 1 to 1000 nm. The term “nano” is simple to define, yet it covers a variety of applications (Figure 1), including several nano-based systems comprised of diverse types of nanocarriers (Figure 2).
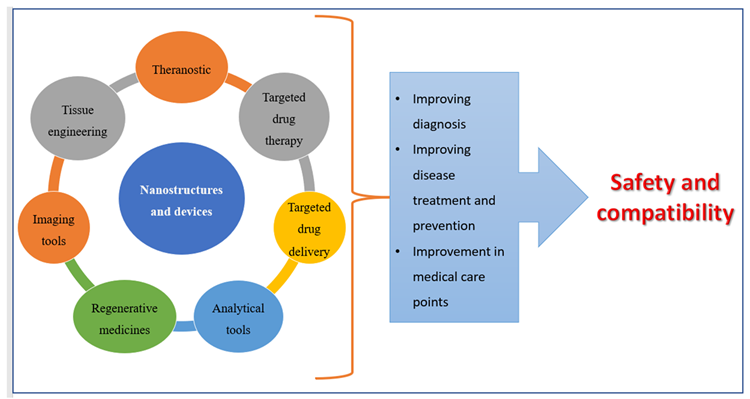
Figure 1. Applications of nanomedicines. Image Credit: Kumari, et al., 2022
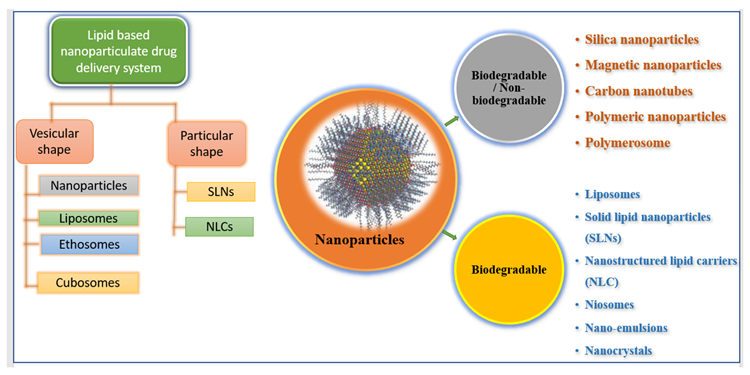
Figure 2. Illustrating various types of nano-formulations. Image Credit: Kumari, et al., 2022
The SLN colloidal medication system, which has particle sizes ranging from 50 to 1000 nm, was developed in the early 1990s. These are emulsifiers that aid in the water stability of a melted solid lipid dispersion. Many processes have been proposed for the manufacture of SLNs (Figure 3), the most common of which being high-pressure homogenization (HPH) and micro-emulsification.
Another study found that “triptolide-loaded SLN” diminished myeloperoxidase (MPO) and glutathione (GSH) activities and functioned as an anti-inflammatory and antioxidant product, resulting in better solubility, reduced toxicity, and lowered GI irritation, as well as ignoring higher local drug concentration and gradual release of drugs. Table 1 contains more examples.
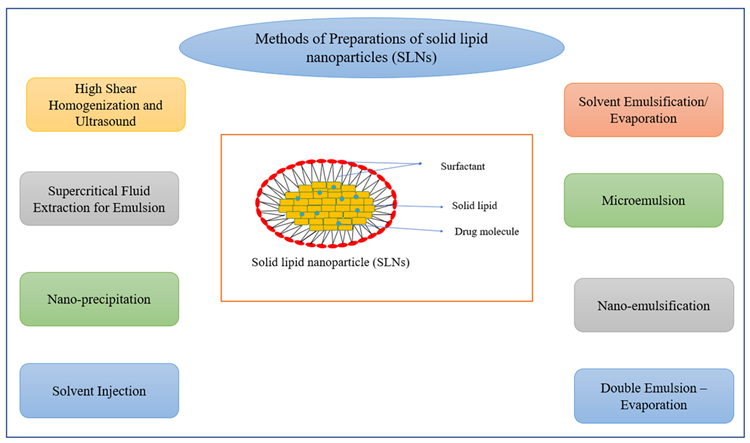
Figure 3. Methods of preparation of solid lipid nanoparticles (SLNs). Image Credit: Kumari, et al., 2022
Table 1. SLN encapsulating natural bioactive. Source: Kumari, et al., 2022
SLNs Loaded with Natural Bioactive |
Plant Source |
Limitations of
Free Drugs |
Advantages of Loaded Drug Molecules |
References |
Triptolide incorporated SLN |
Tripterygium wilfordii Hook F |
Poor water solubility and high toxicity, |
Improved solubility, hyperemia, reduced toxicity, irritation to GIT, etc. |
[33] |
Puerarin-loaded
SLN |
Pueraria lobata (wild) Howe |
Poor water solubility and low oral bioavailability |
3-folds increase in absorption and bioavailability improved tissue concentration in targeted organs (heart and brain) |
[31,32] |
Noscapine PEG conjugated SLN |
Papaveraceae family |
Shorter half-life, less efficacy to glioblastoma cells |
Improved biological half-life, and anticancer efficacy in glioblastoma in vitro and in Swiss male albino mice induced with brain cancer. |
[34] |
Tetrandrine-loaded SLN |
Stephania tetrandra
S. Moore |
Lesser bioavailability and drug release |
Improved bioavailability, in vitro drug release, cellular uptake into human lens epithelial cell line (SRA 01/04) |
[35] |
Cantharidin-loaded SLN |
Mylabris phalerata pallas or mylabris cicchorii linnaeus |
Lesser bioavailability and drug release |
Sustained drug release without a burst effect, improved bioavailability when administered orally in rats induced with gastric mucus membrane irritation. |
[36] |
Hydroxycitric acid-loaded SLN |
Garcinia
cambogia |
Low bioavailability |
Increased bioavailability tested on Wistar rat, anti-obesity medication |
[37] |
Ginkgo biloba leaf extract-loaded SLN |
Ginkgo
biloba |
Low bioavailability |
Improved oral bioavailability at a 5 mg/kg dose, causing blood coagulation at higher doses i.e., 50 mg/kg. |
[38] |
Aloe vera-loaded SLNs |
Aloe vera |
Cause irritation to the skin on multiple uses in some cases |
Incorporated into sunscreen cream, SPF was found to be as per the marketed formulation. |
[39] |
Zataria multiflora essential oil (ZMEO) containing SLN |
Zataria
multiflora |
Mosquito repellant properties at higher doses |
Improved mosquito repellent activities, three times increase in protection time of nano-formulation compared to non-formulated essential oil |
[40] |
Witepsol-loaded SLNs |
Cocoa butter |
Lower
stability |
Suitable vehicle for herbal extracts, higher stability, and proper release profile in the intestine. |
[41] |
Due to their improved regulated drug release, improved drug loading capability, stability, and low drug loss after encapsulation, NLCs beat SLNs.
Several studies looked at the method to entrap bioactives in NLC by changing water solubilities, managing drug release, extending circulation time, co-delivery, drug delivery methods, and improving gastrointestinal absorption and oral bioavailability. In Figure 4, different techniques for preparing NLCs are listed.
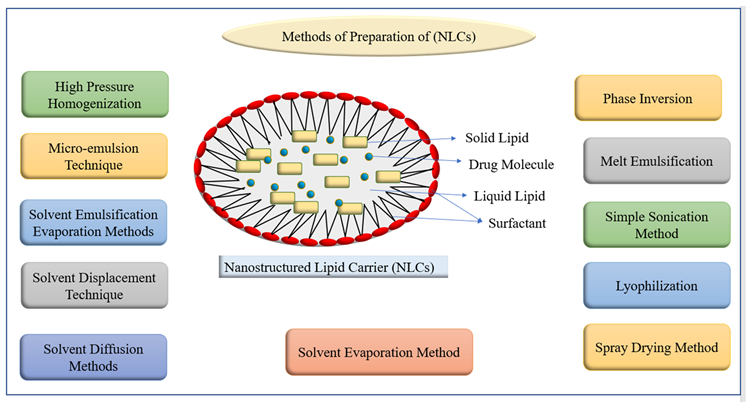
Figure 4. Methods of preparation of nanostructured lipid carriers (NLCs). Image Credit: Kumari, et al., 2022
Table 2. NLCs incorporated bioactives. Source: Kumari, et al., 2022
Drug-Loaded NLCs |
Plant Source |
Limitations of Free Drugs |
Advantages of Loaded Drug Molecules over Conventional Systems |
Reference |
Cardamom essential oil-loaded NLCs |
Elettaria
cardamom |
Low antimicrobial activities |
Protect antimicrobial activity of the plant extract, used as food supplement |
[46] |
Thymoquinone-loaded NLCs |
Nigella
sativa |
Low bioavailability |
Enhanced bioavailability and oral drug delivery, antioxidant potential, improved liver biomarkers affected with PCM induced hepatotoxicity |
[47] |
Citral-loaded
NLCs |
Cymbopogon citratus |
Low
solubility |
Improved water solubility and sustained drug release |
[48] |
β-Elemene incorporated NLCs |
Nigella damascena L. |
Low bioavailability and anticancer efficacy |
Improved bioavailability in male wistar rats and anti-tumor efficacy in H22 hepatoma induced in Kunming mice, reduced venous irritation after i.v., injection in New Zealand white rabbits. |
[49] |
Zerumbone-loaded NLCs |
Zingiber zerumbet
L. Smith |
Low
solubility |
Improved water solubility, bioavailability, and sustained drug release with enhanced anticancer activities both in vitro and in vivo. |
[50,51] |
Baicalin-loaded NLCs |
Scutellaria baicalensis |
Low solubility and bioavailability |
Improved sustained drug release and antidiabetic effect of baicalin |
[52] |
Berberine incorporated NLCs |
Coptis
chinensis |
Low bioavailability |
Enhanced anti-inflammatory potential of the berberine, improved ulcerative colitis symptoms. |
[53] |
Curcumin-loaded NLCs |
Curcuma
longa |
Low solubility and bioavailability |
Improving impressions of DR5 proteins, enhanced caspase 8 and caspase 3 activities, enhanced apoptosis in hepatocellular carcinoma |
[54] |
Hesperidin and clarithromycin-loaded NLCs |
Flavanone
glycoside |
Low bioavailability |
Improved sustained and controlled drug release that can be used to increase the rate of H. pylori eradication. |
[55] |
Diosgenin and Glycyrrhiza glabra extract-loaded NLCs |
Dioscorea deltoideaGlycyrrhiza glabra |
Possessed lessened anti-inflammatory properties |
Inhibition of pro-inflammatory cytokines, TNF-α, IL, and enhanced anti-inflammatory properties |
[56] |
Cinnamaldehyde-loaded (NLC) |
Cinnamomum ceylanicum |
Low bioavailability and shelf life |
Total bacteria and fungi count in the treated CA-loaded NLC samples was about 3.5 log CFU/g less than the control. CA-loaded NLC can extend the shelf life of date fruit without any undesirable impacts on sensory attributes. |
[57] |
Ursolic acid-loaded NLCs |
Pentacyclic terpene acid |
Low
solubility |
Animals infected with Leishmania (Leishmania) infantum and treated with UA-NLC showed lower parasitism than the infected controls, Increased protective immune response, spleen and liver preservation, and the normalization of hepatic and renal functions. |
[58] |
Naringenin (NGN) incorporated NLCs |
Citrus fruits and tomato |
Poor water solubility |
Elevated drug release rate in simulated intestinal solutions in vitro, improved transepithelial transport in MDCK cells, improved oral absorption in mice, enhanced inhibitory effects of NGN on MCD diet-induced mouse NAFLD. |
[59] |
Two approaches for producing nanocrystals have been established: a top-down approach and a bottom-up approach. Precipitation, high gravity-controlled precipitation technology, sono-crystallization, limited impinging liquid jet precipitation method, and multi-inlet vortex mixing procedures are all examples of top-down procedures (Figure 5).
The bottom-up technique, on the other hand, incorporates the use of high-pressure homogenization in grinding activities.
The polymer’s swelling and mucoadhesion properties allowed for continuous drug release, resulting in improved inhalation efficacy. Table 3 contains other examples.
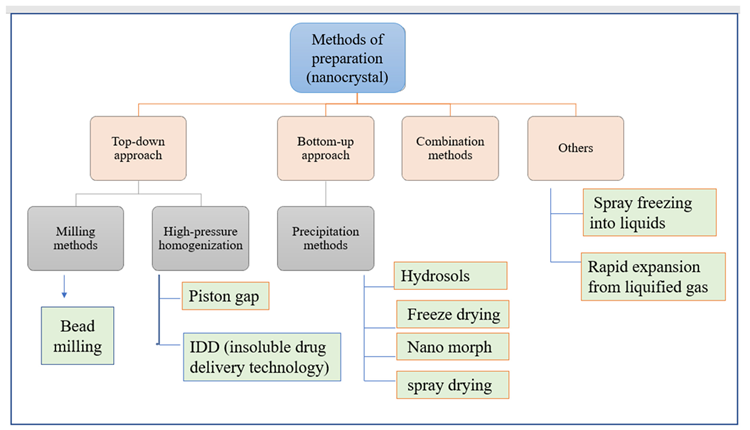
Figure 5. Methods of preparation of nanocrystal. Image Credit: Kumari, et al., 2022
Table 3. NLCs incorporated bioactives. Source: Kumari, et al., 2022
Nanocrystals of
Herbal Compounds |
Plant Source |
Limitations of Free Drugs |
Results and Outcomes of Loaded Formulations |
References |
Rutin incorporated
nanocrystals (RNs) |
Buckwheat, eucalyptus |
Poor water solubility |
Improved water solubility and bioavailability, RNs showed 100 times more cytotoxic effect on HN5 cells, decreased expressions of Bcl-2 mRNA |
[64] |
Cellulose nanocrystals isolated from Amla pomace |
Phyllanthus emblica |
Free drugs do not possess this property |
Cellulose nanocrystals help in converting food industry waste into valuable products, and act as a low-cost precursor for various nanoformulations |
[65] |
Curcumin (CUR) and beclomethasone dipropionate (BDP) nanocrystals |
Curcuma longa |
Poor water solubility and bioavailability |
Improved water solubility and bioavailability, therapeutic efficacy, improved lung delivery of active molecule, improved asthmatic conditions |
[66] |
Silymarin nanocrystals |
Silybum Marianum |
Low
solubility |
Improved drug dissolution profile, sustained drug release |
[67] |
Ethanol extract from Ficus glomerata nanocrystals |
Ficus glomerata |
Lesser biological properties |
Showed comparable activities against Aedes aegypti, Culex quinquefasciatus, and Anopheles stephensi to the conventional neem oil-based nanoemulsion and repellent properties are more effective than commercial formulation. |
[68] |
Puerarin |
Pueraria lobata |
Low bioavailability |
Enhanced oral bioavailability and upgraded brain accumulation for the treatment of Parkinson’s disease (PD) |
[69] |
Resveratrol
nanocrystals |
Natural polyphenol |
Low water solubility |
Improved water solubility and dermal patches preparation for treatments of acne and skin diseases |
[70] |
Nano-emulsions (NE) are non-homogeneous, transparent colloidal dispersion systems with a size of 100 nm that are both optically and thermodynamically stable. These are made up of water and oil, with co-surfactant and surfactant added later.
Figure 6 depicts various NE preparation methods as well as the structure of nanoemulsions. These are concentrated in the lymphatic system, supplied through intramuscular and subcutaneous routes, due to their increased interior membrane permeability.
More illustrations of NE application scenarios are shown in Table 4.
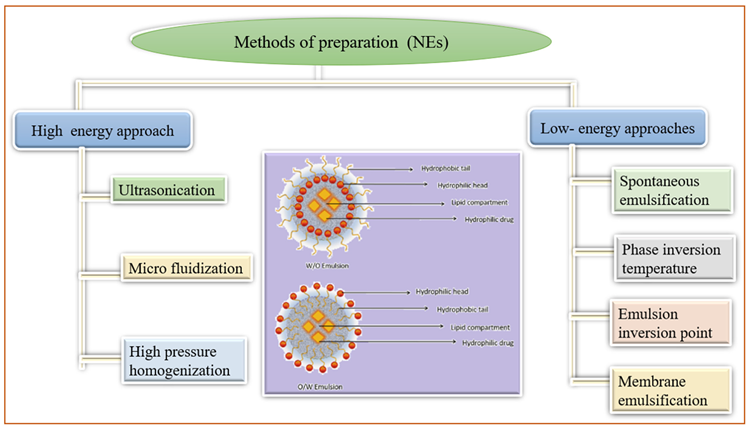
Figure 6. Methods of preparation of nanocrystal. Image Credit: Kumari, et al., 2022
Table 4. NLCs incorporated bioactives. Source: Kumari, et al., 2022
Herbal Nano-Emulsion |
Plant
Source |
Limitations of
Free Drugs |
Results and Outcomes of
Loaded Bioactive |
References |
Hydroxy-safflor yellow A NE |
Carthamus tinctorius |
Low absorption and bioavailability |
Enhanced systemic absorption and improved bioavailability. |
[77] |
Oregano oil NE |
Origanum
vulgare |
Limited spectrum antibiotics |
Reduced and controlled growth of food-borne bacteria (L. monocytogenes, S. Typhimurium, and E. coli) on fresh lettuce. |
[71] |
Elemene oil NE |
Curcuma
species |
Low stability and bioavailability |
Improved stability and oral bioavailability in Sprague Dawley rats than a commercial elemene emulsion. |
[78] |
Quercetin NE |
Many plant parts like nuts |
Low skin penetration cause skin irritation |
Increased cutaneous permeability reached the systemic circulation with lower skin retention. |
[79] |
Basil oil NE |
Ocimum
basilicum |
Have lesser antibacterial activity |
Antibacterial activity against pure E. coli culture |
[80] |
Nigella sativa L. NE |
Nigella
sativa L. |
Limited free radicle scavenging activity |
Enhanced and dose-dependent radical scavenging capacity in the DPPH assay (IC50 of about 47 µg/mL), reduced bioavailability of A2780 cancerous cells, NE showed pro-apoptotic, antioxidant, and anticancer effects. |
[81] |
Linseed oil NE |
Linum usitatissimum
seed |
Poorer stability and penetration through the skin membrane |
Improved stability and physicochemical properties for topical applications, suitable for atopic dermatitis evaluated through in vitro and in silico studies. |
[82] |
Cumin tincture-loaded NE |
Cuminum
cyminum L. |
Limited free radicle scavenging activity and antibacterial properties |
Good and dose-dependent radical scavenging capacity, antioxidant, anti-angiogenic effect, antibacterial activity against S. aureus and K. pneumonia. |
[83] |
Essential oil NE |
Alhagi
maurorum |
Limited bioavailability |
Enhanced antibacterial and antibiofilm activity, identified as antimicrobial agents against antibiotic-resistant bacteria. |
[84] |
Nelumbo nucifera crude extracts. |
Nelumbo
nucifera |
Poorer
stability |
Enhanced stability and antimicrobial activities act as an alternative active ingredient for skin bacterial infection. |
[85] |
Peppermint and rosemary essential oils NE |
Mentha piperita, Mint family Lamiaceae |
Dermal irritation and toxicity |
Reduced osteoarthritis pain via increasing antioxidant capacity and improving the histopathological features of the rats’ knee joint. |
[86] |
Essential oil NE |
Thymus
vulgaris |
Limited antifungal properties |
Obtained as promising alternatives for the treatment of cutaneous mycoses, especially when the etiological agents are resistant to conventional antifungal drugs. |
[87] |
Essential oil NE |
Myristica fragrans or Lavandula dentata |
Poorer
stability |
Improved physical and chemical stability in different temperature and storage conditions |
[88] |
Due to its exceptional attribute of possessing phospholipid bilayers, liposomes can boost drug solubility, drug delivery, the bioavailability of the entrapped drug, uptake of the drug inside a cell, and drug distribution all through the body both in vivo and in vitro. Figure 7 shows the structure of liposomes as well as various liposome production methods.
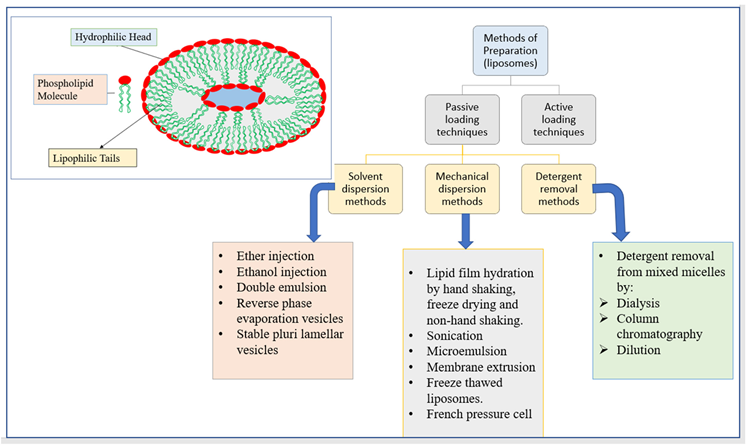
Figure 7. The structure of liposomes and different methods of preparation of liposomes. Image Credit: Kumari, et al., 2022
To develop vaccines, nutraceuticals, and cosmetics, the ADME profiles of medications such as herbs, enzymes, and proteins can be adjusted suitably. Furthermore, unique characteristics such as environmental preservation of the entrapped drug molecule, severe primary destruction of loaded bioactive, cost-effectiveness, and fast therapy with minimal systemic morbidity boosted their utilization in bio-medicine formulations.
More herbal substances encapsulated in liposomes can be found in Table 5.
Table 5. Liposomes containing herbal bioactives. Source: Kumari, et al., 2022
Liposomes of Herbal Compounds |
Plant
Source |
Limitations of Free Drugs |
Results |
Reference |
Baicalin-loaded
liposomes |
Root of Scutellaria baicalensis Georgi) |
Low water solubility and drug release |
Improved solubility, sustained release, enhanced drug concentration in brain tissue after i.v. administration in rats |
[95] |
Polydatin-loaded
liposomes |
Root and rhizome of Polygonum cuspidatum Sieb |
Poorer solubility and bioavailability |
Enhanced oral bioavailability, improved solubility, and sustained release in vitro. |
[96] |
Paclitaxel-loaded/PEGylated/
saturated PC-
based liposomes |
The bark of Taxus brevifolia or pacific yew |
Low solubility and bioavailability |
Improved bioavailability, solubility, biodistribution, and intracellular uptake. |
[97,98] |
Naringenin-loaded
liposomes |
Immature orange fruit and the peels of grapefruits) |
Poorer solubility and bioavailability |
Improved stability, solubility, bioavailability, and tissue distribution the sustained release both in vivo and in vitro after oral administration. |
[99] |
Sterols-loaded
liposomes |
Flammulina
velutipes |
Limited solubility and bioavailability |
Improved water solubility, oral bioavailability, and tissue distribution in liver tumor-bearing Kunming mice. |
[100] |
Quercetin-loaded
liposomes |
Flavonoids |
Reduced solubility and bioavailability |
Improved water solubility, and oral bioavailability, used in wound healing |
[101] |
Curcumin-loaded
liposomes |
Curcuma longa |
Low anticancer properties |
Anticancer and anti-inflammatory potential |
[102] |
Curcumin-loaded
thiolated polymer-
coated liposomes |
Curcuma longa |
Low bioavailability |
The improved therapeutic index of curcumin, Aphthous ulcer |
[103] |
Colchicine-loaded liposomes |
Colchicum autumnal, gloriosa superba extract |
Poorer drug release |
The anti-gout drug, improved drug transport |
[104] |
Liposomal neem gel |
Azadirachta indica leaves |
Limited antibacterial spectrum |
Enhanced anti-bacterial activities |
[105] |
Capsaicin liposomes |
Genus capsicum |
Low bioavailability |
Enhanced bioavailability, treating neuropathic pain |
[106] |
Brucine liposomes |
Nux vomica |
Low bioavailability and showed side effects |
Reduced side effects of brucine like violent seizures |
[107] |
Guggul liposomes |
Commiphora
Mukul. |
Low bioavailability |
Improved anti-inflammatory properties. |
[108] |
Asparagus racemosus liposomes |
Asparagus racemosus |
Low bioavailability |
Improved anti-inflammatory properties. |
[109] |
Polygonum aviculare L. herba (PAH) extract entrapped liposomes quercetin-entrapped liposomes |
Polygonum aviculare
L.Quercetin |
Low cell viability |
Moderately efficient on cell viability while quercetin-loaded liposomes showed increased cell viability and provide better endothelial protection compared to free quercetin and PAH-loaded liposomes |
[110] |
Phytosomes are lipid-compatible molecular aggregates that encase pharmacologically active and water-soluble phytochemicals in phospholipids, increasing solubility and bioavailability. Due to their large molecular size, hydrophilic phytochemicals like polyphenols and flavonoids have reduced absorption in the body, making absorption through biological membranes challenging. Phytosomes have helped to overcome these limitations.
The active material in liposomes is dissolved in the medium contained in the membrane layers, but the active material in phytosomes is an integral part of the membrane (Figure 8).
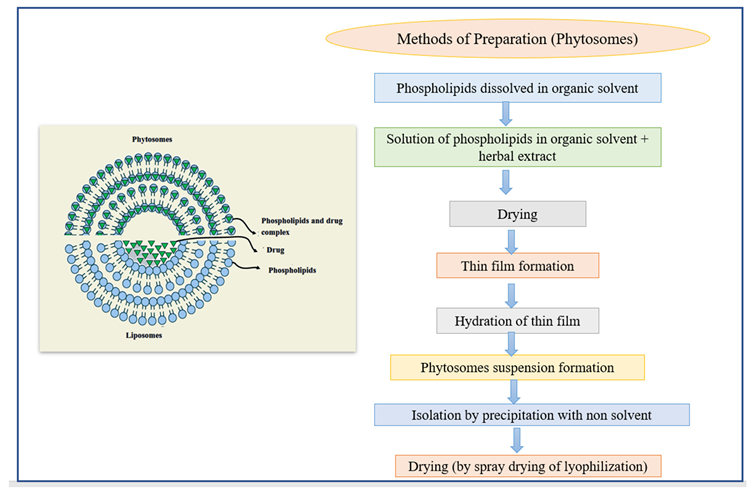
Figure 8. The structure of phytosomes and different methods of the preparation of liposomes. Image Credit: Kumari, et al., 2022
Table 6. Phytosomes containing herbal medicines. Source: Kumari, et al., 2022
Phytosome |
Plant
Source |
Limitations of Free Drugs |
Results and
Outcomes |
Reference |
Epigallocatechin gallate-loaded phytosome |
Camellia sinensis |
Low stability and bioavailability |
Improved solubility and bioavailability. Physicochemical stability through organoleptic, water content, and physicochemical properties at various temperatures |
[114] |
Rutin-loaded phytosome |
Citrus
fruits |
Low stability and poor drug release |
Improved solubility, stability, releasing dynamics and bioavailability in vitro, good antioxidant agent |
[115] |
Soybean seed Phytosome-based thermogel |
Glycine max L. |
Low drug absorption and solubility |
Improved absorption, instability, insolubility, and fast releasing. A clear reduction in body weight, adipose tissue weight, studied in vivo. |
[116] |
Gingerol-loaded phytosome |
Zingiber officinale |
Poor stability and drug absorption |
Improved stability, oral absorption, bioavailability, sustained release, showing potent antioxidant, antibacterial (against Staphylococcus aureus and E. coli), and anti-inflammatory activities in vitro. |
[117] |
Butea monosperma flower extract-loaded phytosome |
Butea monosperma |
Poor water solubility and bioavailability |
Improved solubility, bioavailability, stability, and release dissolution pattern and showed significant free radical scavenging activity in vitro using the DPPH model. |
[118] |
Swertia perennis L.-loaded phytosome |
Swertia perennis L. |
Poor drug release profile. |
Improved entrapment efficiency and in vitro drug release of embedded phytomedicine. |
[119] |
Aloe Vera extract-loaded phytosome |
Aloe Vera |
Limited anticancer activity |
Inhibitory effect on the growth of the MCF-7 cancer cell line, enhanced oral delivery of aloe vera, making its use in cancer therapy. |
[120] |
Morinda lucida extract-loaded phytosome |
Morinda lucida |
Limited antimicrobial activities |
In vivo, anti-plasmodium studies confirmed a higher anti-malarial effect comparable/similar to the standard drug (artesunate). |
[121] |
Aqueous extract of stem bark and lecithin of Tecomella undulata-loaded phytosome |
Tecomella undulata |
Poor drug release profile and bioavailability |
Good entrapment efficiency and drug release in nano sizes (up to 90%), improved bioavailability without resorting to any pharmacological adjuvant or structural modification of the ingredients. |
[122] |
As a result, PEGlycated liposomes were found to be the most effective ex vivo transdermal drug delivery technique, effectively decreasing paw edema in the rat model. Table 7 contains other examples.
Table 7. Ethosomes incorporated with herbal medicines. Source: Kumari, et al., 2022
Herbal Drug-Loaded Ethosomes |
Plant
Source |
Limitations of Free Drugs |
Results and
Outcomes |
References |
Apigenin-loaded ethosomes |
From many fruits and vegetables such as chamomile |
Low bioavailability |
The strong anti-inflammatory activity caused by ultraviolet B light exposure after topical application |
[125] |
Berberis aristata extract-loaded ethosomal gel |
Berberis
aristata |
Lesser drug penetration and bioavailability |
Enhanced permeation profile and transdermal delivery of the extract provide a better approach for dermatological disorders |
[126] |
Cryptotanshinone-loaded ethosomal gel |
Salvia
miltiorrhiza |
Lesser drug penetration and bioavailability |
Enhanced transdermal flux, skin permeation, and deposition on pigskin in vitro. Improved anti-acne activity with reduced skin irritation in the ear of rabbit model associated with ethosomal gel. |
[127] |
Colchicine trans ethosomal gel |
From dried corns and seeds of plants of the genus Colchicum |
Poor stability, solubility drug release bioavailability |
Improved stability, solubility, sustained release, bioavailability, and skin diffusion in vitro.Enhanced drug accretion, tissue biodistribution, and skin permeation in an ex vivo using Sprague Dawley rats’ back skin |
[123] |
Piperine-loaded ethosomes |
Piper nigrum |
Lesser drug penetration and bioavailability |
Ethosomal cream showed higher deposition in skin layers, non-toxic to HaCat cell lines, and novel drug carrier for management of atopic dermatitis. |
[128] |
Achillea millefolium L.-loaded ethosomes |
Achillea millefolium L. |
Limited free radical scavenging activities and drug release |
Enhanced free radical scavenging activities by about 88%, improved drug release by about 79.8% |
[129] |
Sambucus nigra L. Extract-loaded ethosomes |
Sambucus
nigra L. |
Cause skin irritation |
Possessed collagenase inhibition activity, excellent skin compatibility, recognized as a potent cosmeceutical ingredient |
[130] |
Sonication, thin-film hydration, micro fluidization, multiple-membrane extrusion, remote loading, reverse-phase evaporation technique, and bubble method are some of the preparation techniques displayed in Figure 9.
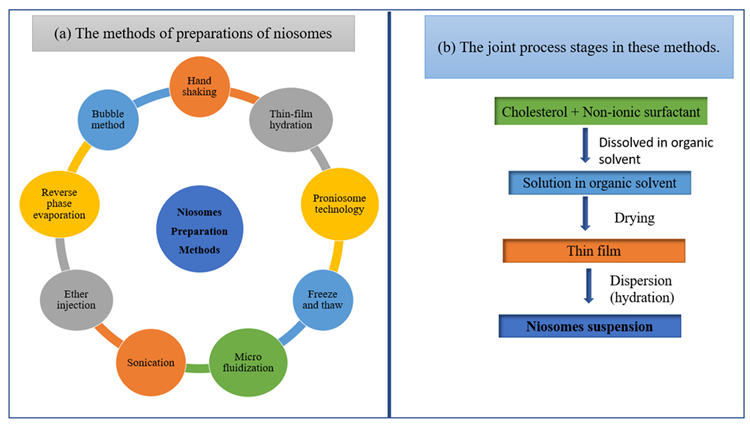
Figure 9. Schematic diagram of (a) the methods of preparations of niosomes and (b) the joint process stages in these methods. Image Credit: Kumari, et al., 2022
Table 8. Niosomes loaded with herbal bioactive. Source: Kumari, et al., 2022
Herbal Medicine-Loaded Niosomes |
Plant
Source |
Limitations of Free Drugs |
Results and
Outcomes |
References |
Permacoce hispida-loaded niosome |
Permacoce hispida- |
Poor stability and bioavailability |
Improved stability, bioavailability, sustained release, and permeability in vitro. Enhanced anti-tuberculosis in vitro. |
[136] |
Embelin-loaded niosome |
Embelia ribes Burm. |
Poor stability and bioavailability |
Improved stability, bioavailability, sustained release, and biocompatibility in vitro. Upgraded streptozotocin-induced diabetes in Albino Wistar rats with potential antioxidant activity. |
[137] |
Lawsone-loaded niosome |
Persian Henna, Lawsonia inermis |
Poor stability and bioavailability |
Improved stability, bioavailability, sustained release, and in vitro permeability. Significantly improved the antitumor activity in MCF-7 cells in vitro. |
[138] |
Rosemarinic acid-loaded niosome |
Rosmarinus officinalis |
Limited drug release and drug stability |
Improved sustained delivery of Niosomal gel of rosmarinic acid to bacteria (Propionibacterium acne and Staphylococcus aureus) infected cells in vitro (anti-acne vulgaris). Improved delivery of naturally occurring antimicrobial and anti-inflammatory agents, in deeper tissues of skin in vivo using Swiss albino mice. |
[139] |
Nerium oleander -loaded niosome |
Nerium
oleander |
Limited antioxidant activity and bioavailability |
Improved cell effectiveness and tolerability of active substances. Improved in vitro cytotoxicity toward cervical and alveolar cancer cells (HeLa and A549) using MTT assay. Displayed potential antioxidant activity in vitro using DPPH radical scavenging assay. |
[140] |
Two techniques have been developed for the manufacture of cubosomes: top-down and bottom-up (Figure 10). Cubosomes have successfully encapsulated somatostatin, indomethacin, insulin, rifampicin, and other drugs.
Instances of cubosomes integrating herbal bioactives are described in Table 9.
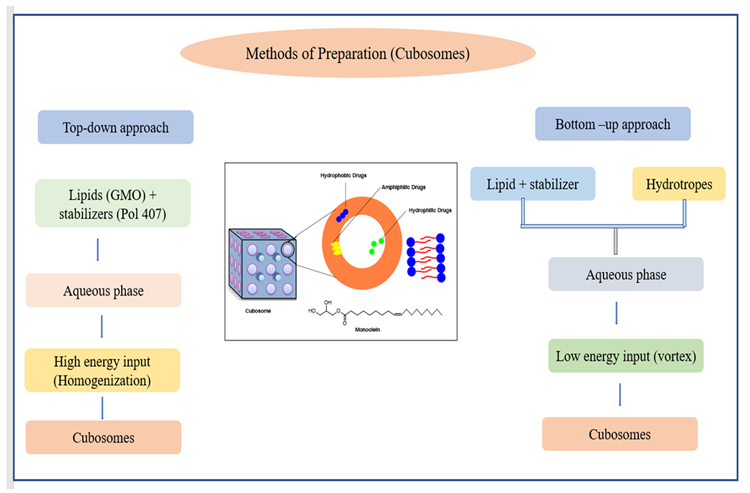
Figure 10. Schematic diagram of (a) the methods of preparations of niosomes and (b) the joint process stages in these methods. Image Credit: Kumari, et al., 2022
Table 9. Niosomes are loaded with herbal bioactive. Source: Kumari, et al., 2022
Herbal Medicine-
Loaded Cubosomes |
Plant
Sources |
Limitations of
Free Drugs |
Results and
Outcomes |
References |
Piperine-loaded cubosomes |
Fruits of the piperaceae family |
Low stability |
Improved stability, hydrophobicity, the enhanced and cognitive effect of piperine, displayed anti-inflammatory, anti-apoptotic, and antioxidant effects. |
[146] |
Curcumin-loaded cubosomes |
Curcuma longa L. |
Low stability |
Upgraded stability, production of nanosized vesicles, and enhanced anti-bacterial properties in topical drug delivery. |
[147] |
Achyranthes bidentata-loaded cubosomes |
Polysaccharides |
Low stability and immunomodulatory effect |
Improved stability, immunomodulatory effect, and displayed fewer toxicities to splenic lymphocytes in vitro. |
[148] |
Capsaicin incorporated cubosomes |
All plants of the capsicum family |
Cause skin
irritation |
Lowered skin irritation, enhanced stability under light and heat, sustained delivery for transdermal administration of capsaicin. |
[149] |
Essential oil of Citrus trifoliata L. incorporated cubosomes |
Citrus trifoliata L. |
Limited insecticidal activities |
Enhanced insecticidal and fungicidal activities against Fusarium oxysporum, Spodoptera littoralis, and Fusarium solani. |
[150] |
Nanotechnology has not only revolutionized medicine, but it has also improved the accuracy and precision with which numerous diseases are treated. It has long been regarded as a superior method for both medication delivery and drug release at the target site.
In addition, tumor killing by heating (hyperthermia), separation and purification of biological molecules and cells, MR imaging contrast enhancement, and phagokinetic investigations are some of the additional medical treatments for cancer diagnosis and therapy.
Nanotechnology allows for advanced therapies with less invasiveness and cost-effective diagnostic equipment that are quicker, lighter, and more sensitive.
The main source of concern is nanoformulation biocompatibility. The simplicity with which nanotechnology-based medicines have been made available at basic levels all across the world and the price of such therapies are the most important factors.
Like any new scientific technique, nanoscience and nanotechnology are the subjects of debate over their utility.
Conclusion
To summarize, pharmaceutical nanotechnology is a rapidly developing branch of science that aims to improve medication stability, solubility, absorption, and bioavailability in inadequately water-soluble and bioavailable pharmaceuticals. Nanotechnology-based solutions also improve the targeted and sustained administration of the entrapped material, resulting in effective treatment potency with fewer adverse effects.
Journal Reference:
Kumari, S., Goyal, A., Gürer, E. S., Yapar, E. A., Garg, M., Sood, M., Sindhu, R. K. (2022) Bioactive Loaded Novel Nano-Formulations for Targeted Drug Delivery and Their Therapeutic Potential. Pharmaceutics, 14(5), p. 1091. Available online: https://www.mdpi.com/1999-4923/14/5/1091/htm.
References and Further Reading
- Atmakuri, L R & Dathi, S (2010) Current trends in herbal medicines. Journal of Pharmacy Research, 3, pp. 109–113.
- Bhokare, S. G., et al. (2016) Herbal novel drug delivery: A review. World Journal of Pharmaceutical Sciences, 5, pp. 593–611. doi.org/10.20959/wjpps20168-7461
- Goldberg, B (1994) lternative Medicine, the Definitive Guide; Future Medicine Publications: Puyallup, WA, USA, 257.
- Patra, J. K., et al. (2018) Nano based drug delivery systems: Recent developments and future prospects. Journal of Nanobiotechnology, 16, p. 71. doi.org/10.1186/s12951-018-0392-8.
- Kulkarni, G T (2011) Herbal drug delivery systems: An emerging area in herbal drug research. Journal of Chronotherapy and Drug Delivery, 2, pp. 113–119.
- Sharma, R., et al. (2017) Nanophytomedicines: A Novel Approach to Improve Drug Delivery and Pharmacokinetics of Herbal Medicine. Bio Bulletin, 3, pp. 132–135.
- Kumar, K & Rai, A (2012) Miraculous therapeutic effects of herbal drugs using novel drug delivery systems. International Research Journal of Pharmacy, 3, pp. 27–30.
- Shah, S. M. A., et al. (2021) Nanobiomedicine: A New Approach of Medicinal Plants and Their Therapeutic Modalities. Journal of Materials and Environmental Science, 12, pp. 1–14.
- Da Silva, P. B., et al. (2014) Nanotechnological strategies for vaginal administration of drugs—A review. Journal of Biomedical Nanotechnology, 10, pp. 2218–2243. doi.org/10.1166/jbn.2014.1890.
- Nune, S. K., et al. (2009) Green nanotechnology from tea: Phytochemicals in tea as building blocks for production of biocompatible gold nanoparticles. Journal of Materials Chemistry, 19, pp. 2912–2920. doi.org/10.1039/B822015H.
- Tapadiya, G G (2017) Impact of nanotechnology on global trade of herbal drugs: An overview. International Journal of Green Pharmacy, 11, p. S171. doi.org/10.22377/IJGP.V11I03.1144
- Shi, X., et al. (2008) Spontaneous formation of functionalized dendrimer-stabilized gold nanoparticles. The Journal of Physical Chemistry C, 112, pp. 8251–8258. doi.org/10.1021/jp801293a.
- Park, S. H., et al. (2006) Loading of gold nanoparticles inside the DPPC bilayers of liposome and their effects on membrane fluidities. Colloids and Surfaces B: Biointerfaces, 48, pp. 112–118. doi.org/10.1016/j.colsurfb.2006.01.006.
- Kabanov, A. V., et al. (2002) Pluronic® block copolymers: Novel functional molecules for gene therapy. Advanced Drug Delivery Reviews, 54, pp. 223–233. doi.org/10.1016/S0169-409X(02)00018-2.
- Mirza, A Z & Siddiqui, F A (2014) Nanomedicine and drug delivery: A mini review. International Nano Letters, 4, p. 94. doi.org/10.1007/s40089-014-0094-7.
- Bairwa, N. K., et al. (2010) Protective effect of stem bark of Ceiba pentandra linn. against paracetamol-induced hepatotoxicity in rats. Pharmacognosy Research, 2, p. 26.
- Wang, N & Feng, Y (2015) Elaborating the role of natural products-induced autophagy in cancer treatment: Achievements and artifacts in the state of the art. BioMed Research International, 2015, p. 934207. doi.org/10.1155/2015/934207.
- Ouattara, B., et al. (1997) Antibacterial activity of selected fatty acids and essential oils against six meat spoilage organisms. International Journal of Food Microbiology, 37, pp. 155–162. doi.org/10.1016/S0168-1605(97)00070-6.
- Sharma, G., et al. (2014) Combinatorial antimicrobial effect of curcumin with selected phytochemicals on Staphylococcus epidermidis. Journal of Asian Natural Products Research, 16, pp. 535–541. doi.org/10.1080/10286020.2014.911289.
- Abdelwahab, S. I. et al. (2013) Thymoquinone-loaded nanostructured lipid carriers: Preparation, gastroprotection, in vitro toxicity, and pharmacokinetic properties after extravascular administration. International Journal of Nanomedicine, 8, p. 2163. doi.org/10.2147/IJN.S44108.
- Paroha, S., et al. (2018) Nanosystems for drug delivery of coenzyme Q10. Environmental Chemistry Letters, 16, pp. 71–77. doi.org/10.1007/s10311-017-0664-9.
- Chandel, A K S & Bhingradiya, N (2021) Therapeutic Efficacy of Herbal Formulations Through Novel Drug Delivery Systems. In Enhancing the Therapeutic Efficacy of Herbal Formulations; IGI Global: Hershey, PA, USA, pp. 1–42. doi.org/10.4018/978-1-7998-4453-2.ch001.
- Sahu, A N (2013) Nanotechnology in herbal medicines and cosmetics. International Journal of Research in Ayurveda and Pharmacy, 4, pp. 472–474. doi.org/10.7897/2277-4343.04334.
- ud Din, F., et al. (2017) Effective use of nanocarriers as drug delivery systems for the treatment of selected tumors. International Journal of Nanomedicine, 12, p. 7291. doi.org/10.2147/IJN.S146315.
- Lam, P. L., et al. (2017) Recent advances in green nanoparticulate systems for drug delivery: Efficient delivery and safety concern. Nanomedicine, 12, pp. 357–385. doi.org/10.2217/nnm-2016-0305.
- Liu, Y & Feng, N (2015) Nanocarriers for the delivery of active ingredients and fractions extracted from natural products used in traditional Chinese medicine (TCM). Advances in Colloid and Interface Science, 221, pp. 60–76. doi.org/10.1016/j.cis.2015.04.006.
- Nagalingam, A (2017) Drug delivery aspects of herbal medicines. Japanese Kampo Medicines for the Treatment of Common Diseases: Focus on Inflammation, 17, p. 143. doi.org/10.1016/B978-0-12-809398-6.00015-9.
- Lin, C. H., et al. (2017) Recent advances in oral delivery of drugs and bioactive natural products using solid lipid nanoparticles as the carriers. Journal of Food and Drug Analysis, 25, pp. 219–234. doi.org/10.1016/j.jfda.2017.02.001.
- Yingchoncharoen, P., et al. (2016) Lipid-based drug delivery systems in cancer therapy: What is available and what is yet to come. Pharmacological Reviews, 68, pp. 701–787. doi.org/10.1124/pr.115.012070.
- Rostami, E., et al. (2014) Drug targeting using solid lipid nanoparticles. Chemistry and Physics of Lipids, 181, pp. 56–61. doi.org/10.1016/j.chemphyslip.2014.03.006.
- Luo, C. F., et al. (2011) Pharmacokinetics, tissue distribution and relative bioavailability of puerarin solid lipid nanoparticles following oral administration. International Journal of Pharmaceutics, 410, pp. 138–144. doi.org/10.1016/j.ijpharm.2011.02.064.
- Luo, C. F., et al. (2013) Metabolic profile of puerarin in rats after intragastric administration of puerarin solid lipid nanoparticles. International Journal of Nanomedicine, 8, p. 933. doi.org/10.2147/IJN.S39349.
- Zhang, C., et al. (2013) Preparation and optimization of triptolide-loaded solid lipid nanoparticles for oral delivery with reduced gastric irritation. Molecules, 18, pp. 13340–13356. doi.org/10.3390/molecules181113340.
- Madan, J., et al. (2013) Poly (ethylene)-glycol conjugated solid lipid nanoparticles of noscapine improve biological half-life, brain delivery and efficacy in glioblastoma cells. Nanomedicine: Nanotechnology, Biology and Medicine, 9, pp. 492–503. doi.org/10.1016/j.nano.2012.10.003.
- Li, J., et al. (2014) Preparation and evaluation of charged solid lipid nanoparticles of tetrandrine for ocular drug delivery system: Pharmacokinetics, cytotoxicity and cellular uptake studies. Drug Development and Industrial Pharmacy, 40, pp. 980–987. doi.org/10.3109/03639045.2013.795582.
- Rajput, S. B., et al. (2013) Anti-Candida properties of asaronaldehyde of Acorus gramineus rhizome and three structural isomers. Chinese Medicine, 8, p. 18. doi.org/10.1186/1749-8546-8-18.
- Shende, P & Narvenker, R (2020) Herbal nanotherapy: A new paradigm over conventional obesity treatment. Journal of Drug Delivery Science and Technology, 61, p. 102291. doi.org/10.1016/j.jddst.2020.102291.
- Jammanesh, A., et al. (2021) Formulation, Characterization and Toxicity Assessment of Ginkgo Biloba Extract Solid Lipid Nanoparticle in female mice. Nanomedicine Research Journal, 6, pp. 28–40.
- Rodrigues, L R & Jose, J (2020) Exploring the photo protective potential of solid lipid nanoparticle-based sunscreen cream containing Aloe vera. Environmental Science and Pollution Research, 27, pp. 20876–20888. doi.org/10.1007/s11356-020-08543-4.
- Kelidari, H. R., et al. (2021) Solid-lipid nanoparticles (SLN) s containing Zataria multiflora essential oil with no-cytotoxicity and potent repellent activity against Anopheles stephensi. Journal of Parasitic Disease, 45, pp. 101–108. doi.org/10.1007/s12639-020-01281-x.
- Campos, D. A., et al. (2015) Stability of bioactive solid lipid nanoparticles loaded with herbal extracts when exposed to simulated gastrointestinal tract conditions. Food Research International, 78, pp. 131–140. doi.org/10.1016/j.foodres.2015.10.025.
- Motawea, A., et al. (2021) Crucial Role of PLGA Nanoparticles in Mitigating the Amiodarone-Induced Pulmonary Toxicity. International Journal of Nanomedicine, 16, p. 4713.
- Das, S., et al. (2012) Are nanostructured lipid carriers (NLCs) better than solid lipid nanoparticles (SLNs): Development, characterizations and comparative evaluations of clotrimazole-loaded SLNs and NLCs? European Journal of Pharmaceutical Sciences, 47, pp. 139–151. doi.org/10.1016/j.ejps.2012.05.010.
- Muhammad, H. S. R. (2014) Anti-Leukemic Effects of Zerumbone Nanoparticle on Human Jurkat T Lymphoblastoid Cell Lines In Vitro and Murine Leukemic WEHI-3B Model In Vivo; Universiti Putra Malaysia Seri Kembangan: Selangor, Malaysia.
- Shangguan, M., et al. (2014) Binary lipids-based nanostructured lipid carriers for improved oral bioavailability of silymarin. Journal of Biomaterials Applications, 28, pp. 887–896. doi.org/10.1177%2F0885328213485141.
- Nahr, F. K., et al. (2018) Food grade nanostructured lipid carrier for cardamom essential oil: Preparation, characterization and antimicrobial activity. Journal of Functional Foods, 40, pp. 1–8. doi.org/10.1016/j.jff.2017.09.028.
- Ong, Y. S., et al. (2018) Thymoquinone loaded in nanostructured lipid carrier showed enhanced anticancer activity in 4T1 tumor-bearing mice. Nanomedicine, 13, pp. 1567–1582. doi.org/10.2217/nnm-2017-0322.
- Nordin, N., et al. (2018) Characterization and toxicity of citral incorporated with nanostructured lipid carrier. PeerJournals, 6, p. e3916. doi.org/10.7717/peerj.3916.
- Jaiswal, M., et al. (2015) Nanoemulsion: An advanced mode of drug delivery system. Biotechnology, 5, pp. 123–127. doi.org/10.1007/s13205-014-0214-0.
- Mohamad, N. E., et al. (2015) Nanostructured lipid carrier improved in vivo anti-tumor and immunomodulatory effect of Zerumbone in 4T1 challenged mice. RSC Advances, 5, pp. 22066–22074. doi.org/10.1039/C5RA00144G.
- Rahman, H.S., et al. (2014) Zerumbone induces G2/M cell cycle arrest and apoptosis via mitochondrial pathway in Jurkat cell line. Natural Product Communications, 9, p. 1934578X1400900904. doi.org/10.1177%2F1934578X1400900904.
- Shi, F., et al. (2016) Nanostructured lipid carriers loaded with baicalin: An efficient carrier for enhanced antidiabetic effects. Pharmacognosy Magazine, 12, p. 198. doi.org/10.4103%2F0973-1296.186347.
- Deng, J., et al. (2020) Berberine-loaded nanostructured lipid carriers enhance the treatment of ulcerative colitis. International Journal of Nanomedicine, 15, p. 3937. doi.org/10.2147/IJN.S247406.
- Wang, F., et al. (2020) Curcumin-loaded nanostructured lipid carrier induced apoptosis in human HepG2 cells through activation of DR5/caspases-mediated extrinsic apoptosis pathway. Acta Pharmaceutica, 70, pp. 227–237. doi.org/10.2478/acph-2020-0003.
- Sharaf, M., et al. (2021) Co-delivery of hesperidin and clarithromycin in a nanostructured lipid carrier for the eradication of Helicobacter pylori in vitro. Bioorganic chemistry, 112, p. 104896. doi.org/10.1016/j.bioorg.2021.104896.
- Lacatusu, I., et al. (2021) Multifaced Role of Dual Herbal Principles Loaded-Lipid Nanocarriers in Providing High Therapeutic Efficacity. Pharmaceutics, 13, p. 1511. doi.org/10.3390/pharmaceutics13091511.
- Akhavan, H. R., et al. (2021) Cinnamaldehyde-Loaded Nanostructured Lipid Carriers Extend the Shelf Life of Date Palm Fruit. Food Bioprocess Technology, 14, pp. 1478–1489. doi.org/10.1007/s11947-021-02645-8.
- Jesus, J. A., et al. (2021) Preclinical Assessment of Ursolic Acid Loaded into Nanostructured Lipid Carriers in Experimental Visceral Leishmaniasis. Pharmaceutics, 13, p. 908. doi.org/10.3390/pharmaceutics13060908.
- Hu, R., et al. (2021) Study on the Inhibitory Effects of Naringenin-Loaded Nanostructured Lipid Carriers Against Nonalcoholic Fatty Liver Disease. Journal of Biomedical Nanotechnology, 17, pp. 942–951. doi.org/10.1166/jbn.2021.3077.
- Junyaprasert, V B & Morakul, B (2015) Nanocrystals for enhancement of oral bioavailability of poorly water-soluble drugs. Asian Journal of Pharmaceutical Sciences, 10, pp. 13–23. doi.org/10.1016/j.ajps.2014.08.005.
- Du, J., et al. (2015) Nanosuspensions of poorly water-soluble drugs prepared by bottom-up technologies. International Journal of Pharmaceutics, 495, pp. 738–749. doi.org/10.1016/j.ijpharm.2015.09.021.
- Pardhi, V. P., et al. (2018) Nanocrystals: An overview of fabrication, characterization and therapeutic applications in drug delivery. Current Pharmaceutical Design, 24, pp. 5129–5146. dx.doi.org/10.2174/1381612825666190215121148.
- Ni, R., et al. (2017) Nanocrystals embedded in chitosan-based respirable swellable microparticles as dry powder for sustained pulmonary drug delivery. European Journal of Pharmaceutical Sciences, 99, pp. 137–146. doi.org/10.1016/j.ejps.2016.12.013.
- Bohlouli, S., et al. (2021) Preparation, Characterization, and Evaluation of Rutin Nanocrystals as an Anticancer Agent against Head and Neck Squamous Cell Carcinoma Cell Line. Journal of Nanomaterials, 2021, p. 9980451. doi.org/10.1155/2021/9980451.
- Gupta, V., et al. (2021) Isolation and characterization of cellulose nanocrystals from amla (Phyllanthus emblica) pomace. Biomass Conversion and Biorefinery, pp. 1–12. doi.org/10.1007/s13399-021-01852-9.
- Casula, L., et al. (2021) Pulmonary Delivery of Curcumin and Beclomethasone Dipropionate in a Multicomponent Nanosuspension for the Treatment of Bronchial Asthma. Pharmaceutics, 13, p. 1300. doi.org/10.3390/pharmaceutics13081300.
- El-Batal, A. I., et al. (2018) Preparation and characterization of silymarin nanocrystals and phytosomes with investigation of their stability using gamma irradiation. Indian Journal of Pharmaceutical Education and Research, 52, pp. 174–183. doi.org/10.5530/ijper.52.4s.96.
- Nazeer, A. A., et al. (2019) Evaluation of larvicidal and repellent activity of nanocrystal emulsion synthesized from F. glomerata and neem oil against mosquitoes. Journal of Cluster Science, 30, pp. 1649–1661. doi.org/10.1007/s10876-019-01611-x.
- Xiong, S., et al. (2019) Oral delivery of puerarin nanocrystals to improve brain accumulation and anti-parkinsonian efficacy. Molecular Pharmaceutics, 16, pp. 1444–1455. doi.org/10.1021/acs.molpharmaceut.8b01012.
- Karakucuk, A & Tort, S (2020) Preparation, characterization and antimicrobial activity evaluation of electrospun PCL nanofiber composites of resveratrol nanocrystals. Pharmaceutical Development and Technology, 25, pp. 1216–1225. doi.org/10.1080/10837450.2020.1805761.
- Vickers, N J (2017) Animal communication: When i’m calling you, will you answer too? Current Biology, 27, pp. R713–R715. doi.org/10.1016/j.cub.2017.05.064.
- Lovelyn, C & Attama, A A (2011) Current state of nanoemulsions in drug delivery. Journal of Biomaterials and Nanobiotechnology, 2, p. 626. dx.doi.org/10.4236/jbnb.2011.225075.
- Mahato, R (2017) Nanoemulsion as targeted drug delivery system for cancer therapeutics. Journal of Pharmaceutical Sciences and Pharmacology, 3, pp. 83–97. doi.org/10.1166/jpsp.2017.1082.
- Kotta, S., et al. (2012) Exploring oral nanoemulsions for bioavailability enhancement of poorly water-soluble drugs. Expert Opinion on Drug Delivery, 9, pp. 585–598. doi.org/10.1517/17425247.2012.668523.
- Khani, S., et al. (2016) Design and evaluation of oral nanoemulsion drug delivery system of mebudipine. Drug Delivery, 23, pp. 2035–2043. doi.org/10.3109/10717544.2015.1088597.
- Patel, R P & Joshi, J R (2012) An overview on nanoemulsion: A novel approach. International Journal of Pharmaceutical Sciences and Research, 3, p. 4640.
- Qi, J., et al. (2011) Enhanced effect and mechanism of water-in-oil microemulsion as an oral delivery system of hydroxysafflor yellow A. International Journal of Nanomedicine, 6, p. 985. doi.org/10.2147/IJN.S18821.
- Zeng, Z., et al. (2010) Preparation, characterization and relative bioavailability of oral elemene o/w microemulsion. International Journal of Nanomedicine, 5, p. 567. doi.org/10.2147/IJN.S12485.
- Bonifácio, B. V., et al. (2014) Nanotechnology-based drug delivery systems and herbal medicines: A review. International Journal of Nanomedicine, 9, p. 1. doi.org/10.2147%2FIJN.S52634.
- Gosh, V., et al. (2013) Ultrasonic emulsifivation of food-grade nanoemulsion formulation and evulation of its bactericial activity. Ultrason. Sono-Chemistry, 20, pp. 338–344. doi.org/10.1016/j.ultsonch.2012.08.010.
- Arazmjoo, S., et al. (2021) Evaluation of anti-cancer and antioxidant properties of nanoemulsions synthesized by Nigella sativa L. tincture. Nanomedicine Journal, 8, 57–64.
- Kildaci, L., et al. (2021) Linseed Oil Nanoemulsions for Treatment of Atopic Dermatitis Disease: Formulation, Characterization, In Vitro and In Silico Evaluations. Journal of Drug Delivery Science and Technology, 64, p. 102652. doi.org/10.1016/j.jddst.2021.102652.
- Asgari, H. T., et al. (2021) Anti-angiogenic, antibacterial, and antioxidant activities of nanoemulsions synthesized by Cuminum cyminum L. tinctures. Journal of Food Measurement and Characterization volume, 15, pp. 3649–3659. doi.org/10.1007/s11694-021-00947-1.
- Hassanshahian, M., et al. (2020) Formulation and characterization of nanoemulsion from Alhagi maurorum essential oil and study of its antimicrobial, antibiofilm, and plasmid curing activity against antibiotic-resistant pathogenic bacteria. Journal of Environmental Health Science & Engineering, 18, pp. 1015–1027. doi.org/10.1007/s40201-020-00523-7.
- Pengon, S., et al. (2020) Development of Antimicrobial Nanoemulsions Containing Nelumbo nucifera Extract. Key Engineering Materials, 859, pp. 226–231. doi.org/10.4028/www.scientific.net/KEM.859.
- Mohammadifar, M., et al. (2021) Anti-osteoarthritis potential of peppermint and rosemary essential oils in a nanoemulsion form: Behavioral, biochemical, and histopathological evidence. BMC Complementary Medicine and Therapies, 21, p. 57. doi.org/10.1186/s12906-021-03236-y.
- Moazeni, M., et al. (2021) In vitro antifungal activity of Thymus vulgaris essential oil nanoemulsion. Journal of Herbal Medicine, 28, p. 100452. doi.org/10.1016/j.hermed.2021.100452.
- Cossetin, L. F., et al. (2021) Development of nanoemulsions containing Lavandula dentata or Myristica fragrans essential oils: Influence of temperature and storage period on physical-chemical properties and chemical stability. Industrial Crops and Products, 160, p. 113115. doi.org/10.1016/j.indcrop.2020.113115.
- Ganesan, P & Narayanasamy, D (2017) Lipid nanoparticles: Different preparation techniques, characterization, hurdles, and strategies for the production of solid lipid nanoparticles and nanostructured lipid carriers for oral drug delivery. Sustainable Chemistry and Pharmacy, 6, pp. 37–56. doi.org/10.1016/j.scp.2017.07.002.
- Robson, A. L., et al. (2018) Advantages and limitations of current imaging techniques for characterizing liposome morphology. Frontiers in Pharmacology, 9, p. 80. doi.org/10.3389/fphar.2018.00080.
- Song, Z., et al. (2017) Cyclic RGD peptide-modified liposomal drug delivery system for targeted oral apatinib administration: Enhanced cellular uptake and improved therapeutic effects. International Journal of Nanomedicine, 12, p. 1941. doi.org/10.2147/IJN.S125573.
- Hong, S. S., et al. (2015) Effects of triglycerides on the hydrophobic drug loading capacity of saturated phosphatidylcholine-based liposomes. International Journal of Pharmaceutics, 483, pp. 142–150. doi.org/10.1016/j.ijpharm.2015.02.013.
- Aqil, F., et al. (2013) Bioavailability of phytochemicals and its enhancement by drug delivery systems. Cancer Letters, 334, pp. 133–141. doi.org/10.1016/j.canlet.2013.02.032.
- Thangapazham, R. L., et al. (2008) Evaluation of a nanotechnology-based carrier for delivery of curcumin in prostate cancer cells. International Journal of Oncology, 32, pp. 1119–1123. doi.org/10.3892/ijo.32.5.1119.
- Li, N., et al. (2018) Preparation, characterization, pharmacokinetics and biodistribution of baicalin-loaded liposome on cerebral ischemia-reperfusion after iv administration in rats. Molecules, 23, p. 1747. doi.org/10.3390/molecules23071747.
- Wang, X., et al. (2015) Novel nanoliposomal delivery system for polydatin: Preparation, characterization, and in vivo evaluation. Drug Design, Development and Therapy, 9, p. 1805. doi.org/10.2147%2FDDDT.S77615.
- Hong, S. S., et al. (2016) Development of paclitaxel-loaded liposomal nanocarrier stabilized by triglyceride incorporation. International Journal of Nanomedicine, 11, p. 4465. doi.org/10.2147%2FIJN.S113723.
- Kannan, V., et al. (2015) Optimization of drug loading to improve physical stability of paclitaxel-loaded long-circulating liposomes. Journal of Liposome Research, 25, pp. 308–315. doi.org/10.3109/08982104.2014.995671.
- Wang, Y., et al. (2017) Enhanced solubility and bioavailability of naringenin via liposomal nanoformulation: Preparation and in vitro and in vivo evaluations. Aaps Pharmscitech, 18, pp. 586–594. doi.org/10.1208/s12249-016-0537-8.
- Yi, C., et al. (2013) Enhanced oral bioavailability and tissue distribution of a new potential anticancer agent, Flammulina velutipes sterols, through liposomal encapsulation. Journal of Agricultural and Food Chemistry, 61, pp. 5961–5971. doi.org/10.1021/jf3055278.
- Jangde, R & Singh, D (2016) Preparation and optimization of quercetin-loaded liposomes for wound healing, using response surface methodology. Artificial Cells, Nanomedicine, and Biotechnology, 44, pp. 635–641. doi.org/10.3109/21691401.2014.975238.
- Jadia, R., et al. (2018) Liposomes aid curcumin’s combat with cancer in a breast tumor model. Oncomedicine, 3, pp. 94–109.
- Kongkaneramit, L., et al. (2016) Development of curcumin liposome formulations using polyol dilution method. Songklanakarin Journal of Science and Technology, 38, pp. 605–610.
- Singh, H. P., et al. (2009) Elastic liposomal formulation for sustained delivery of colchicine: In vitro characterization and in vivo evaluation of anti-gout activity. The AAPS Journal, 11, pp. 54–64. doi.org/10.1208/s12248-008-9078-8.
- Singh, A., et al. (2014) Design, development and characterization of liposomal neem gel. International Journal of Pharmaceutical Sciences and Research, 5, pp. 140–148.
- Zhu, Y., et al. (2015) Improved oral bioavailability of capsaicin via liposomal nanoformulation: Preparation, in vitro drug release and pharmacokinetics in rats. Archives of Pharmacal Research, 38, pp. 512–521. doi.org/10.1007/s12272-014-0481-7.
- Qin, X.-q., et al. (2007) Preparation of liposomal brucine and its pharmaceutical/pharmacodynamic characterization. Acta Pharmaceutica Sinica B, 28, pp. 1851–1858. doi.org/10.1111/j.1745-7254.2007.00654.x.
- Paliwal, S., et al. (2011) Synergistic effect of guggul liposomes lipid nano-vesicles for the treatment of inflammation. International Journal of Life Sciences and Biotechnology, 6, pp. 1–13.
- Plangsombat, N., et al. (2016) Anti-inflammatory activity of liposomes of Asparagus racemosus root extracts prepared by various methods. Experimental and Therapeutic Medicine, 12, pp. 2790–2796. doi.org/10.3892/etm.2016.3661.
- Mureşan, M., et al. (2021) Comparative Study of the Pharmacological Properties and Biological Effects of Polygonum aviculare L. herba Extract-Entrapped Liposomes versus Quercetin-Entrapped Liposomes on Doxorubicin-Induced Toxicity on HUVECs. Pharmaceutics, 13, p. 1418. doi.org/10.3390/pharmaceutics13091418.
- Suryawanshi, J S (2011) Phytosome: An emerging trend in herbal drug treatment. Journal of. Medical Genetics and Genomics, 3, pp. 109–114.
- Neelam, K., et al. (2012) Various Techniques For The Modification Of Starch And The Applications Of Its Derivatives. International Research Journal of Pharmacy, 3, pp. 25–31.
- Karthivashan, G., et al. (2016) Optimization, formulation, and characterization of multiflavonoids-loaded flavanosome by bulk or sequential technique. International Journal of Nanomedicine, 11, p. 3417. doi.org/10.2147/IJN.S112045.
- Anwar, E & Farhana, N (2018) Formulation and Evaluation of Phytosome-Loaded Maltodextrin-Gum Arabic Microsphere System for Delivery of Camellia sinensis Extract. Journal of Young Pharmacists, 10, pp. S56–S62. doi.org/10.5530/jyp.2018.2s.11.
- Hooresfand, Z., et al. (2015) Preparation and characterization of rutin-loaded nanophytosomes. Journal of Pharmaceutical Sciences, 21, pp. 145–151. .
- El-Menshawe, S. F., et al. (2018) Nanosized soy phytosome-based thermogel as topical anti-obesity formulation: An approach for acceptable level of evidence of an effective novel herbal weight loss product. International Journal of Nanomedicine, 13, p. 307. doi.org/10.2147/IJN.S153429.
- Singh, R. P., et al. (2018) Phytosome complexed with chitosan for gingerol delivery in the treatment of respiratory infection: In vitro and in vivo evaluation. European Journal of Pharmaceutical Sciences, 122, pp. 214–229. doi.org/10.1016/j.ejps.2018.06.028.
- Gahandule, M., et al. (2016) Formulation and development of hepato-protective butea monosperma-phytosome. International Journal of Research in Pharmaceutical Sciences, 1, pp. 21–27.
- Chouhan, A., et al. (2021) Formulation, development and evaluation of phytosomes of swertia perennis. Asian Journal of Pharmaceutical Education and Research, 10, pp. 55–56. doi.org/10.38164/AJPER/10.2.2021.55-66.
- Murugesan, M. P., et al. (2021) Evaluation of anti-cancer activity of phytosomes formulated from aloe vera extract. Materials Today: Proceedings, 42, pp. 631–636. doi.org/10.1016/j.matpr.2020.11.047.
- Calister, U., et al. (2020) Formulation and evaluation of Morinda lucida based-phytosome complexes for malaria treatment. Technology, 6, p. 9.
- Nagpal, N., et al. (2016) Designing of a phytosome dosage form with Tecomella undulata as a novel drug delivery for better utilization. Pakistan Journal of Pharmaceutical Sciences, 29, pp. 1231–1236.
- Abdulbaqi, I. M., et al. (2018) Transethosomal gels as carriers for the transdermal delivery of colchicine: Statistical optimization, characterization, and ex vivo evaluation. Drug Design, Development and Therapy, 12, p. 795. doi.org/10.2147/DDDT.S158018.
- Zhao, Y. Z., et al. (2013) Selection of high efficient transdermal lipid vesicle for curcumin skin delivery. International Journal of Pharmaceutics, 454, pp. 302–309. doi.org/10.1016/j.ijpharm.2013.06.052.
- Khaled, S.A., et al. (2014) Desktop 3D printing of controlled release pharmaceutical bilayer tablets. International Journal of Pharmaceutics, 461, pp. 105–111. doi.org/10.1016/j.ijpharm.2013.11.021.
- Fatima, Z (2017) Formulation and Performance evaluation of Berberis aristata extract loaded ethosomal gel. Asian Journal of Pharmaceutics, 11, p. 1401. doi.org/10.22377/ajp.v11i03.1401.
- Yu, Z., et al. (2016) Ethosomes loaded with cryptotanshinone for acne treatment through topical gel formulation. PLoS ONE, 11, p. e0159967. doi.org/10.1371/journal.pone.0159967.
- Kumar, P., et al. (2021) Topical creams of piperine loaded lipid nanocarriers for management of atopic dermatitis: Development, characterization, and in vivo investigation using BALB/c mice model. Journal Liposome Research, 32, pp. 62–73. doi.org/10.1080/08982104.2021.1880436.
- Andleeb, M., et al. (2021) Development, Characterization and Stability Evaluation of Topical Gel Loaded With Ethosomes Containing Achillea millefolium L. Extract. Frontiers in Pharmacology, 12, p. 336. doi.org/10.3389/fphar.2021.603227.
- Mota, A. H., et al. (2021) A Newfangled Collagenase Inhibitor Topical Formulation Based on Ethosomes with Sambucus nigra L. Extract. Pharmaceuticals, 14, p. 467. doi.org/10.3390/ph14050467.
- Nagalakshmi, S., et al. (2016) Fabrication and characterization of herbal drug-loaded nonionic surfactant based niosomal topical gel. Journal of Pharmaceutical Sciences and Research, 8, p. 1271.
- Seleci, D. A., et al. (2015) Phyto-Niosomes: In Vitro Assessment of the Novel Nanovesicles Containing Marigold Extract. International Journal of Polymeric Materials and Polymeric Biomaterials, 64, pp. 927–937.
- Rohilla, R., et al. (2016) Herbal and polymeric approaches for liver-targeting drug delivery: Novel strategies and their significance. Drug Delivery, 23, pp. 1645–1661. doi.org/10.3109/10717544.2014.945018.
- Ambwani, S., et al. (2018) Current knowledge on nanodelivery systems and their beneficial applications in enhancing the efficacy of herbal drugs. Journal of Experimental Biology and Agricultural Sciences, 6, pp. 87–107. doi.org/10.18006/2018.6(1).87.107.
- Rameshk, M., et al. (2018) Proliferation and in vitro wound healing effects of the Microniosomes containing Narcissus tazetta L. bulb extract on primary human fibroblasts (HDFs). DARU Journal of Pharmaceutical Sciences, 26, pp. 31–42. doi.org/10.1007/s40199-018-0211-7.
- Anghore, D & Kulkarni, G T (2017) Development of novel nano niosomes as drug delivery system of spermacoce hispida extract and in vitro antituberculosis activity. Current Nanomaterials, 2, pp. 17–23. doi.org/10.2174/2405461502666170314151949.
- Scognamiglio, I., et al. (2013) Nanocarriers for topical administration of resveratrol: A comparative study. International Journal of Pharmaceutics, 440, pp. 179–187. doi.org/10.1016/j.ijpharm.2012.08.009.
- Barani, M., et al. (2018) Lawsone-loaded Niosome and its antitumor activity in MCF-7 breast Cancer cell line: A Nano-herbal treatment for Cancer. DARU Journal of Pharmaceutical Sciences, 26, pp. 11–17. doi.org/10.1007/s40199-018-0207-3.
- Budhiraja, A & Dhingra, G (2015) Development and characterization of a novel antiacne niosomal gel of rosmarinic acid. Drug Delivery, 22, pp. 723–730. doi.org/10.3109/10717544.2014.903010.
- Kaur, H., et al. (2017) Synthesis and characterization of ZIF-8 nanoparticles for controlled release of 6-mercaptopurine drug. Journal of Drug Delivery Science and Technology, 41, pp. 106–112. doi.org/10.1016/j.jddst.2017.07.004.
- Rizwan, S B & Boyd, B J (2015) Cubosomes: Structure, preparation and use as an antigen delivery system. In Subunit Vaccine Delivery; Springer: Berlin/Heidelberg, Germany, pp. 125–140. doi.org/10.1007/978-1-4939-1417-3_7.
- Garg, M., et al. (2021) An Update on the Recent Advances in Cubosome: A Novel Drug Delivery System. Current Drug Metabolism, 22, pp. 441–450. doi.org/10.2174/1389200221666210105121532.
- Tian, Y., et al. (2017) Folic acid-targeted etoposide cubosomes for theranostic application of cancer cell imaging and therapy. Medical Science Monitor : International Medical Journal of Experimental and Clinical Research, 23, p. 2426. doi.org/10.12659/MSM.904683.
- Azmi, I. D., et al. (2015) Cubosomes and hexosomes as versatile platforms for drug delivery. Therapeutic Delivery, 6, pp. 1347–1364. doi.org/10.4155/tde.15.81.
- Bazylińska, U., et al. (2018) Polymer-free cubosomes for simultaneous bioimaging and photodynamic action of photosensitizers in melanoma skin cancer cells. Journal of Colloid and Interface Science, 522, pp. 163–173. doi.org/10.1016/j.jcis.2018.03.063.
- Elnaggar, Y. S., et al. (2015) Novel piperine-loaded Tween-integrated monoolein cubosomes as brain-targeted oral nanomedicine in Alzheimer’s disease: Pharmaceutical, biological, and toxicological studies. International Journal of Nanomedicine, 10, p. 5459. doi.org/10.2147/IJN.S87336.
- Archana, A., et al. (2015) Curcumin loaded nano cubosomal hydrogel: Preparation, in vitro characterization and antibacterial activity. Chemical Science Transactions, 4, pp. 75–80. doi.org/10.2147/IJN.S87336.
- Ou, N., et al. (2018) Evaluation of optimum conditions for Achyranthes bidentata polysaccharides encapsulated in cubosomes and immunological activity in vitro. International Journal of Biological Macromolecules, 109, pp. 748–760. doi.org/10.1016/j.ijbiomac.2017.11.064.
- Peng, X., et al. (2015) Characterization of cubosomes as a targeted and sustained transdermal delivery system for capsaicin. Drug Design, Development and Therapy, 9, p. 4209. doi.org/10.2147/DDDT.S86370.
- Abdel-Kawy, M. A., et al. (2021) Chemical composition and potentiation of insecticidal and fungicidal activities of Citrus trifoliata L. fruits essential oil against Spodoptera littoralis, Fusarium oxysporum and Fusarium solani via nano-cubosomes. Natural Product Research. 35, pp. 2438–2443. doi.org/10.1080/14786419.2019.1675063.
- Mahtab, R., et al. (1995) Protein-sized quantum dot luminescence can distinguish between “straight”, “bent”, and “kinked” oligonucleotides. Journal of the American Chemical Society, 117, pp. 9099–9100. doi.org/10.1021/ja00140a040.
- Ma, J., et al. (2003) Biomimetic processing of nanocrystallite bioactive apatite coating on titanium. Nanotechnology, 14, pp. 619–623.
- Yoshida, J & Kobayashi, T (1999) Intracellular hyperthermia for cancer using magnetite cationic liposomes. Journal of Magnetism and Magnetic Materials, 194, pp. 176–184. doi.org/10.1016/S0304-8853(98)00586-1.
- Molday, R S & MacKenzie, D (1982) Immunospecific ferromagnetic irondextran reagents for the labeling and magnetic separation of cells. Journal of Immunological Methods, 52, pp. 353–367. doi.org/10.1016/0022-1759(82)90007-2.
- Weissleder, R., et al. (1990) Ultrasmall superparamagnetic iron oxide: Characterization of a new class of contrast agents for MR imaging. Radiology, 175, pp. 489–493. doi.org/10.1148/radiology.175.2.2326474.
- Parak, W. J., et al. (2002) Cell motility and metastatic potential studies based on quantum dot imaging of phagokinetic tracks. Advance Materials, 14, pp. 882–885. doi.org/10.1002/1521-4095(20020618)14:12%3C882::AID-ADMA882%3E3.0.CO;2-Y.
- Salata, O (2004) Applications of nanoparticles in biology and medicine. Journal Nanobiotechnology, 2, p. 3. doi.org/10.1186/1477-3155-2-3.
- Alok, A., et al. (2013) Nanotechnology: A boon in oral cancer diagnosis and therapeutics. SRM journal of research in dental sciences, 4, pp. 154–160. doi.org/10.4103/0976-433X.125591.