Nanoelectronics – in which semiconductor components’ critical features such as logic transistors and memory measure well under 100 nm – is a new and rapidly growing field. Potential applications in quantum computing, advanced memory, and energy storage and generation make this a tiny technology with enormous potential.
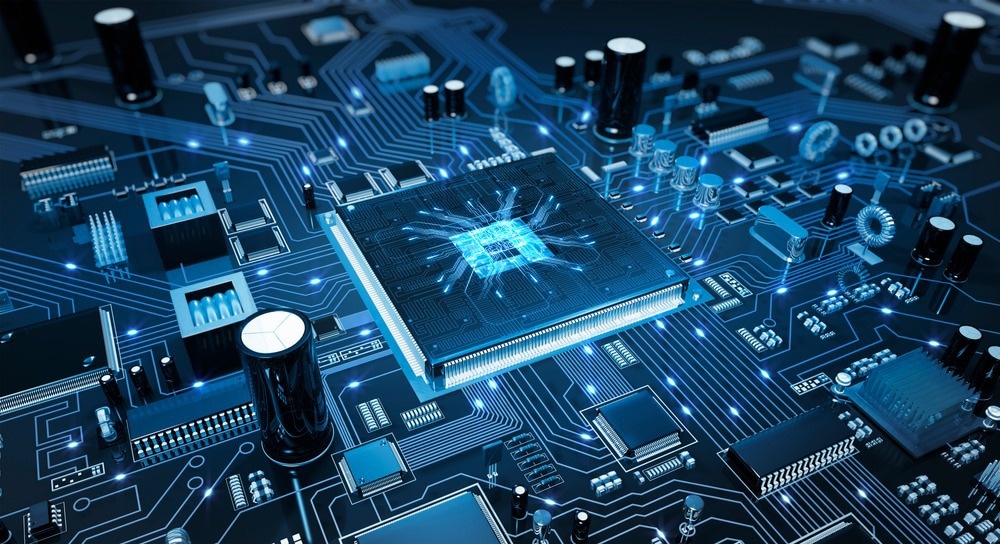
Image Credit: NIMEDIA/Shutterstock.com
A History of Nanoelectronics
The first nanoscale electronic devices were developed by researchers in the 1960s, who produced gold thin film just 10 nm in thickness as the base for a metal semiconductor junction transistor.
In the late 1980s, a team of IBM researchers demonstrated the first metal oxide semiconductor field effect transistor (MOSFET) with a gate oxide thickness of just 10 nm. The product used tungsten gate technology to achieve this nanoscale dimension.
The first multi-gate MOSFET, the FinFET, was developed in 1989. The FinFET, or fin field effect transistor, is a double gate MOSFET that is also three-dimensional and non-planar. In 2002, a 10 nm FinFET was fabricated.
A CMOS (complementary MOS) transistor was developed in 1999 to show just how far MOSFET transistor technology could take us in terms of nanoscale electronics.
Just a few years later, in 2006, researchers developed a MOSFET measuring just 3 nm, making it the smallest nanoelectronic device in the world at the time.
Nanoelectronic semiconductor devices went into commercial production in the 2010s, and Samsung is currently releasing a 3 nm GAAFET, or gate all round FET on the market.
How Was Nanoelectronics Made Possible?
Nanoscale electronic devices are the culmination – or, more accurately, the latest product – of decades of cutting-edge research in nanosciences and nanotechnology.
Ever since Richard Feynman proposed the possibility of computing with “submicroscopic” computers in a groundbreaking lecture in 1959, researchers at the forefront of physics, materials science, and instrumentation design and manufacturing development have been pursuing ever smaller microscopic measurements.
This journey has led to a number of groundbreaking applications in information and communication technology (ICT) – personal computers, smartphones, Internet of Things (IoT) technology, and many more everyday game changers of the modern world rely on progress gained by nanoelectronic research.
But truly, nanoelectronic devices are yet to reach our shelves.
While semiconductor technology has progressed remarkably, even microscopic computers are still mostly out of reach. Sub microscopic (nanoscale) computers may be even farther off.
Current semiconductor technology may even be limited in terms of minimum system sizes, and we may be approaching that limit.
However, nanoelectronics currently under development may enable us to break this barrier by developing and manufacturing truly sub-microscopic, nanoscale electronic devices in the next few decades.
Moving Nanoelectronics Forward
Researchers propose that the best way to develop nanoelectronics is to combine microelectronic devices with nanoelectronics devices in hybrid systems.
This approach builds on the progress already achieved in microelectronics, for example, in developing microelectromechanical systems (MEMS) technology which has brought numerous MEMS sensors like accelerometers and microphones, magnetometers and gyroscopes, and even power generators to the market.
One current innovation pursuing this hybrid approach is a solid state quantum effect nanoelectronic device for resonance tunneling. The device uses a standard silicon bulk effect transistor to generate a multi-state switching device, which its developers refer to as a “resonance tunneling transistor.”
The resonance tunneling transistor can be used for making circuitry with greater available logic density than conventional microelectronic transistor logic is capable of.
Another nanoelectronic device in development is the single electron transistor or SET. The SET is a switching device that controls electron tunneling and uses this to amplify a current.
Two tunnel junctions, each made of two pieces of metal and a sub-nanometer thin insulator between them, share a common electrode. Electrons must tunnel through the insulator material to get between electrodes.
Because quantum tunneling is a discrete process, the electric charge produced by electrons moving through the tunnel junction is produced in multitudes of each electron’s charge.
Devices like electronic tunneling devices, as well as quantum dots, work with quantized energy. This is energy in its smallest possible interacting parts, at a scale where quantum physics phenomena like particle entanglement, tunneling, and particle superposition can be observed.
Nanoelectronics devices will deploy electrons over incredibly small regions. Naturally, energy quantization and its effects on the devices and their intended functions are significant research focuses at the moment.
Another focus of research in the nanoelectronics field is investigating ways to use electrically conductive polymers in nanoscale applications of organic electronics.
Researchers are studying electrically conductive nanostructured polymers, nanoparticle-based polymers, and polymer nanocomposites dispersed with conductive nanoparticles.
These materials are optimal for creating nanoscale electronic devices that are organic. This is due to the nanopolymers’ suitability as a building block material for both complicated and simple hierarchical nanostructures.
Organic nanoelectronics and nanostructured electronic systems can also be used in conjunction with π-conjugated polymers to act as electron acceptors in next-generation organic nanoscale photovoltaic devices.
References and Further Reading
Achilli, S., et al. (2021). Position-Controlled Functionalization of Vacancies in Silicon by Single-Ion Implanted Germanium Atoms. Advanced Functional Materials. doi.org/10.1002/adfm.202011175.
Altawell, N. (2022). Nanoelectronic systems. Introduction to Machine Olfaction Devices. doi.org/10.1016/B978-0-12-822420-5.00014-3.
Khalifeh, S. (2020). Optimized Electronic Polymers, Small Molecules, Complexes, and Elastomers for Organic Electronic Systems. Polymers in Organic Electronics. doi.org/10.1016/B978-1-927885-67-3.50008-0.
Shilov, A. (2019). Samsung Completes Development of 5nm EUV Process Technology. [Online] Anand Tech. Available at: https://www.anandtech.com/show/14231/samsung-completes-development-of-5-nm-euv-process-technology
Tian, B., et al (2007). Coaxial silicon nanowires as solar cells and nanoelectronic power sources. Nature. doi.org/10.1038/nature06181.
Zhirnov, V.V., and R.K. Cavin III (2015). The nanomorphic cell: atomic-level limits of computing. Microsystems for Bioelectronics. doi.org/10.1016/B978-0-323-31302-5.00001-6.
Disclaimer: The views expressed here are those of the author expressed in their private capacity and do not necessarily represent the views of AZoM.com Limited T/A AZoNetwork the owner and operator of this website. This disclaimer forms part of the Terms and conditions of use of this website.