Learn about how nanoscale approaches are impacting the development and efficacy of vaccine technologies.
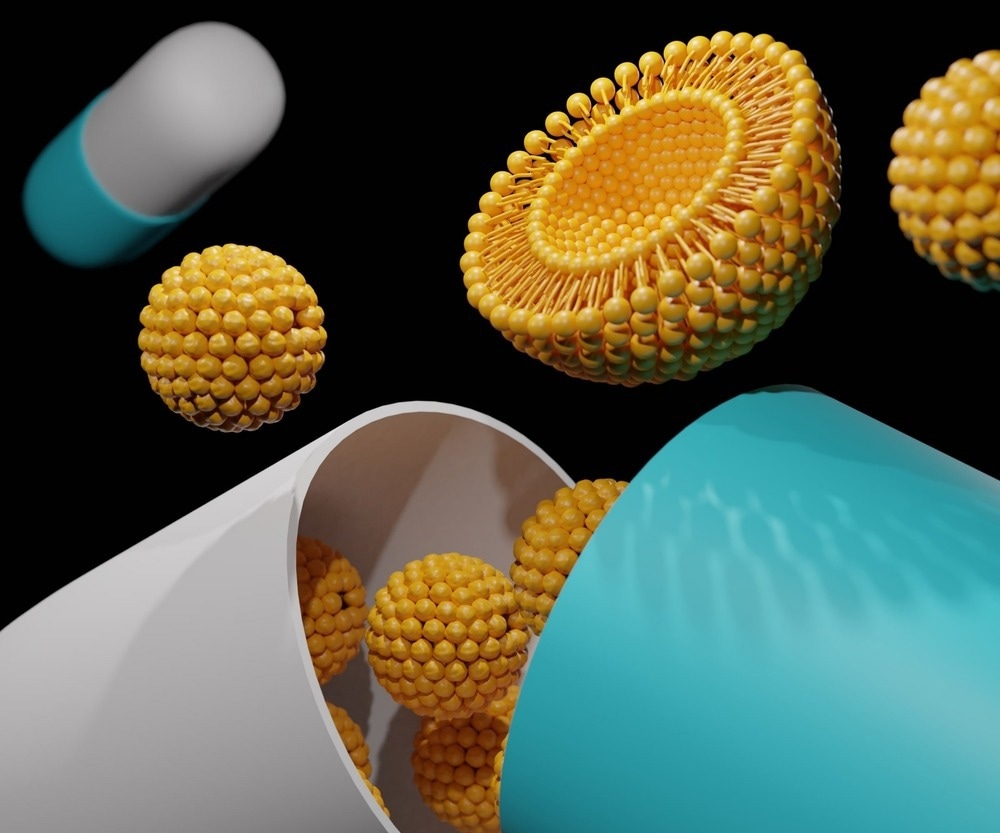
Image Credit: Love Employee/Shutterstock.com
The development of vaccines has enabled the prevention of several diseases, such as diphtheria, rubella, measles, tetanus, yellow fever pertussis, and Haemophilus influenzae type b (Hib) disease. Nanotechnology has revolutionized vaccination research by offering greater effectiveness and a safe platform. To date, several nanoscale vaccines have been developed to prevent different infections and malignancies. The newly developed nanovaccines based on mRNA technology exhibited improved innate and adaptive immunity. These vaccines are also known as next-generation vaccines.1
Evolution in Vaccine Development Methods
Historically, vaccines were developed using live attenuated organisms, inactivated toxins, or killed whole organisms. Live attenuated vaccines (e.g., polio, varicella, and measles) induced both humoral and cellular immunity. However, this vaccine type has been associated with a serious risk of reverting to its virulent form. The other vaccine types, i.e., toxoid and whole organism vaccines, induce a weaker immune response and require multiple booster doses.
Advanced technologies, such as recombinant DNA technology, have been used to develop subunit and conjugate vaccines, which induce stronger immune responses against weak antigens. DNA vaccines contain genes encoding a particular antigen(s). Although DNA vaccines have so far only received approval for veterinary use, in vitro experimental results exhibited their potential against many human diseases, such as tuberculosis and human immunodeficient virus-1 (HIV-1).2 Subunit vaccines contain a portion of a pathogen or a protein that acts as an antigen and induces an immune response.
The key advantages of subunit and conjugate vaccines are increased safety and lack of contaminants. Both vaccines can be produced in large quantities using recombinant methods. Although these vaccines are effective, they require a carrier for efficient delivery and adjuvants. The main function of an adjuvant is to enhance immune response. Studies have shown that an immunogenic antigen acts more efficiently when loaded into an efficient delivery system.
Delivery vehicles and adjuvants protect antigens from degradation.3 At present, very few vaccine adjuvants have received approval for use in humans. Although both aluminum salts and MF59 are the two most popular vaccine adjuvants, the mode of action as adjuvants remains unclear.
Development of Nanoscale Vaccines
Different types of nanomaterials are selected based on their size, composition, and surface properties to develop vaccines or nanocarriers. Lipid-based nanoparticles, polymeric nanoparticles, protein nanoparticles, biomimetic nanoparticles, and inorganic nanocarriers are commonly used for vaccine development. Some of the different types of nanomaterials used for nanovaccines are discussed below:
Protein Nanoparticles:
Many protein nanoparticles have been derived from natural sources and are used to deliver antigens. Since natural nanomaterials exhibit superior biodegradability and biocompatibility, self-assembled protein nanoparticles are excellent candidates for nanovaccines. Virus-like particles (VLP), pyruvate dehydrogenase, and ferritin family proteins have shown significant promise in developing nanovaccines.4 At present, VLPs-based vaccines, namely, Sci-B-Vac™ against hepatitis virus, and Cervarix® and Gardasil® against human papillomavirus virus (HPV) are available commercially.5
Polymeric Nanoparticles:
The polymeric nanoparticles are solid, can be self-adjuvant, and their size ranges between 10 and 1000 nm. These nanoparticles have exhibited high immunogenicity and stability. Both natural polymeric nanomaterials (e.g., dextran and dextran) and synthetic polymeric nanomaterials (e.g., poly lactic acid) are excellent nanovaccines candidates.
Chitosan is a natural polymer possessing cationic charge and bioadhesive properties, making it a suitable candidate for gene delivery. These nanoparticles are used as coating agents to improve adherence and immunogenicity.6
Lipid-based Nanoparticles:
Lipid nanoparticles (LNPs) are developed through self-assembling of amphipathic phospholipid molecules. These nanoscale lipid vesicles are potential nanocarriers for nucleic acid delivery. Ionizable lipids and cationic lipids are suitable components of LNPs. Some of the key advantages of LNPs include high biocompatibility, controlled release properties, and low toxicity.
LNPs are extensively used in mRNA-based vaccines owing to their ability to trigger immune response. Many preclinical and clinical studies have indicated the potential of LNPs for nanovaccine development. It must be noted that LNPs have been used in the development of mRNA-based coronavirus disease 2019 (COVID-19) vaccines, namely, mRNA-1273 and BNT162b2.7,8
Inorganic Nanomaterials:
Inorganic materials, such as inorganic salts, metals and oxides, and non-metal oxides, are used in nanomedicine. These materials exhibited low biodegradability but high structural stability. Therefore, inorganic nanomaterials are modified to improve biocompatibility before utilizing it in nanovaccines. Gold, iron, and silica nanoparticles are commonly used for antigen delivery.
Gold nanoparticles have high antigen loading capacity, low immunogenicity, and suitable biocompatibility. Furthermore, they exhibit intrinsic immunostimulatory effects with the capacity to trigger the production of inflammatory cytokines. Therefore, gold nanoparticles have dual functions, i.e., antigen carriers and triggering of immune responses.9 Owing to suitable pore size and morphology, silica nanoparticles can be used as nanovaccines carrier materials.
Biomimetic Nanomaterials:
Biomimetic nanomaterials can efficiently perform complex biofunctions. These have been recently exploited in nanovaccines development, particularly for efficient delivery to the target site. Biomimetic nanomaterials can interact with targeted biological systems.
Simple biomimetic nanoparticles use natural ligands or peptides (e.g., candoxin and arginylglycylaspartic acid) to enhance efficient delivery. The vaccine components are often camouflaged with cell membranes to avoid the immune system and target lesions. Virosome is an emerging biomimetic nanoparticle exploited to develop nanovaccines against viral infection.10
Carbon-based Nanomaterials:
Carbon-based nanomaterials and carbon nanotubes have been recently assessed for antigen delivery systems. For instance, a purified tuberculin protein derivative was loaded into a carboxyl group-functionalized single-walled carbon nanotubes (SWCNTs) via covalent binding. A subcutaneous introduction of these nanovaccines induced an immune response using Th1 cells of cytokines.11
Future of Nanoscale Vaccines
Millions of people across the world have received multiple doses of COVID-19 vaccines. Compared to conventional vaccine technologies, the nanoscale lipid-based and adenovirus-based vaccine vectors can be developed more quickly and have exhibited higher effectiveness in preventing severe infection.
It is important to understand the duration of protection against infection offered by the nanovaccines. To develop nanoscale vaccines effective against new coronavirus variants, it is vital to assess the ease and flexibility of modifying the original nanoscale vaccine design. The fate of future nanovaccines is also dependent on the manufacturing cost, handling ease, and logistics, i.e., storage, maintenance, and stability, of thermolabile molecules (RNA, lipids).12
References and Further Reading
Omidi, Y., et al. (2021) Nanoscale Vaccines: Design, Delivery, and Applications. pp. 437-468. doi.org/10.1002/9783527832095.ch14
Peek, L. J., et al. (2008) Nanotechnology in vaccine delivery. Advanced Drug Delivery Reviews. 60(8), pp. 915-928. doi.org/10.1016/j.addr.2007.05.017
Hutcheon, C. J., et al. (2006) Physiochemical and functional characterization of antigen proteins eluted from aluminum hydroxide adjuvant. Vaccine. 24, pp. 7214–7225.
Feng, C., et al. (2022) Emerging vaccine nanotechnology: From defense against infection to sniping cancer. Acta Pharmaceutica Sinica B., 12(5), pp. 2206-2223. doi.org/10.1016/j.apsb.2021.12.021
National Cancer Institute (2023) Human Papillomavirus (HPV) Vaccines. Available at: https://www.cancer.gov/about-cancer/causes-prevention/risk/infectious-agents/hpv-vaccine-fact-sheet. [Assessed on January 3, 2023.]
Wu, D. J., et al. (2020) Chitosan-based colloidal polyelectrolyte complexes for drug delivery: a review. Carbohydrate Polymers. 238, p. 116126.
Anderson, E. J., et al. (2020) Safety and immunogenicity of SARS-CoV-2 mRNA-1273 vaccine in older adults. The New England Journal of Medicine. 383, pp. 2427-2438.
Khurana, A., et al. (2021) Role of nanotechnology behind the success of mRNA vaccines for COVID-19. Nano Today. 38, p. 101142
Tang, Z. M., et al. (2020) A materials-science perspective on tackling COVID-19. Nature Reviews Materials. 5, pp. 847-860.
Felnerova, D., et al. (2004) Liposomes and virosomes as delivery systems for antigens, nucleic acids and drugs. Current Opinion in Biotechnology. 15, pp. 518-529.
Kim, M., et al. (2014) Nanotechnology and vaccine development. AJPS. 9(5), pp. 227-235. doi.org/10.1016/j.ajps.2014.06.002
Kisby, T., et al. (2021) Reasons for success and lessons learnt from nanoscale vaccines against COVID-19. Nature Nanotechnology 16(8), pp. 843-850. doi.org/10.1038/s41565-021-00946-9
Disclaimer: The views expressed here are those of the author expressed in their private capacity and do not necessarily represent the views of AZoM.com Limited T/A AZoNetwork the owner and operator of this website. This disclaimer forms part of the Terms and conditions of use of this website.