By Owais AliReviewed by Lexie CornerSep 25 2024
The drug development process is notoriously lengthy, expensive, and prone to failure during later stages of clinical trials. Traditional 2D cell culture models, while helpful, often fail to accurately replicate the complex 3D tissue environment, leading to misleading drug efficacy and toxicity results and higher development costs.
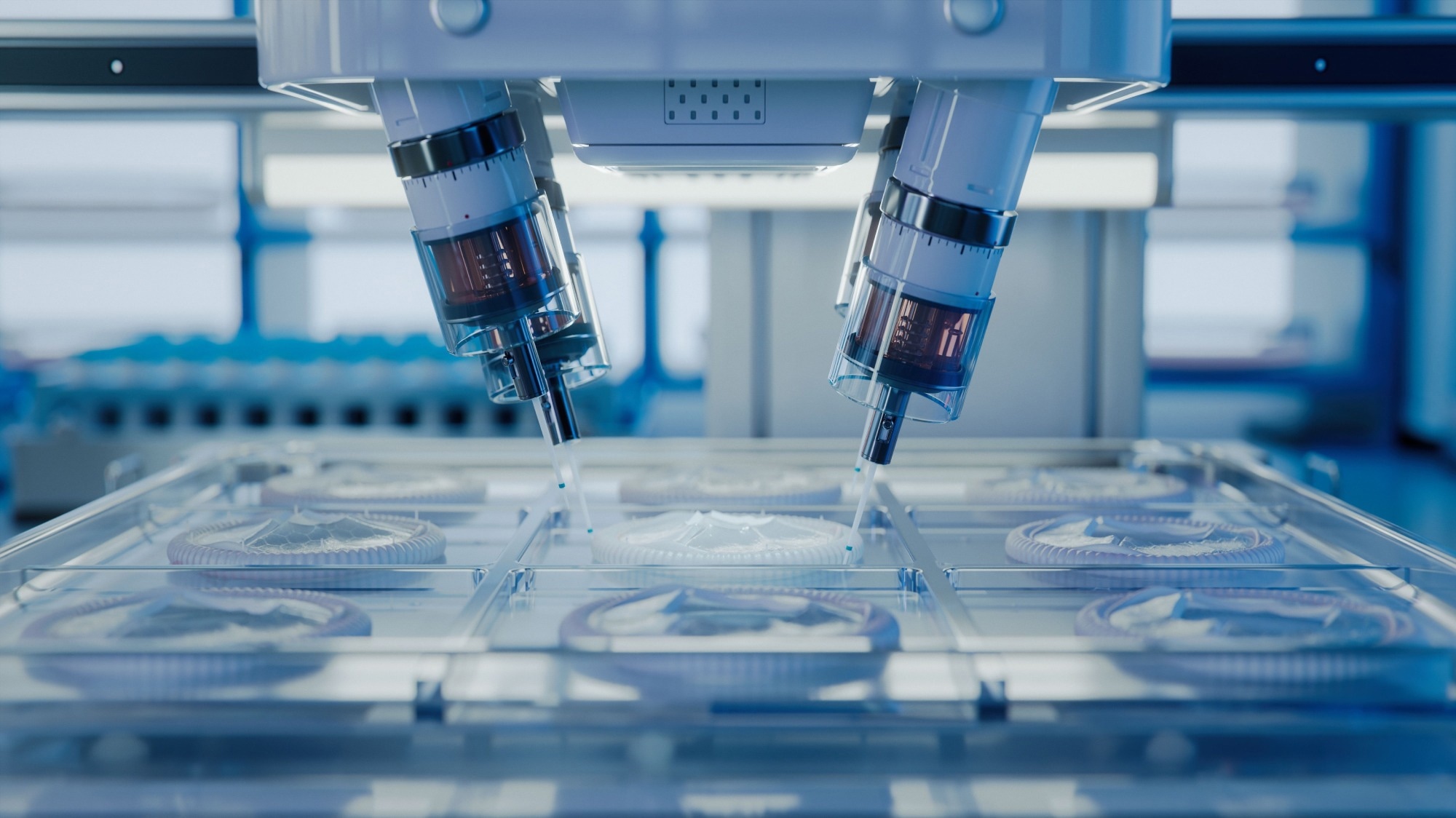
Image Credit: IM Imagery/Shutterstock.com
Recently, nanoparticle-driven 3D bioprinting has emerged as a revolutionary approach for high-throughput drug screening, offering enhanced scalability, accuracy, and efficiency while reducing reliance on animal testing.
Role of Nanoparticle-Enhanced 3D Bioprinting in Drug Testing
Nanoparticle-driven 3D bioprinting is an innovative approach that combines the precision of 3D printing with the use of biocompatible nanoparticles, such as iron oxide and gold, to create complex, multicellular 3D structures. These nanoparticles can be controlled by external stimuli, like magnetic fields or light, to guide cells into organized 3D arrangements.
The process involves suspending cells and nanoparticles in a biocompatible hydrogel matrix, which, when extruded through a 3D printer, allows the nanoparticles to precisely position the cells. This high level of control enables researchers to create tissue models that closely replicate the architecture and cellular organization of native tissues.
These detailed models provide a more accurate in vivo representation of tissue environments, especially for drug testing, allowing researchers to assess drug efficacy, toxicity, and potential therapeutic responses more effectively.1
For example, Inventia and Revvity recently developed an automated workflow using the RASTRUM™ high-throughput bioprinter to streamline drug discovery and testing with 3D culture assays.
This system uses tissue-mimicking hydrogels to create 3D cell cultures, enabling precise, large-scale formation in microplates. It supports high-throughput screening with more physiologically relevant drug response predictions compared to traditional 2D or spheroid models.2
Advantages of Nanoparticle-driven 3D bioprinting in Drug Screening Processes
Nanoparticle-driven 3D bioprinting technology significantly enhances the accuracy, efficiency, and scalability of drug screening processes by simplifying tissue fabrication and facilitating extensive studies of cell behavior.
Unlike traditional 2D cell cultures, which often fail to replicate the intricate cell-microenvironment interactions and can lead to discrepancies in drug efficacy, 3D bioprinted models provide a more accurate representation of in vivo cell microenvironment, including multiple cell types, extracellular matrix (ECM), and nutrient exchange pathways.
The drug development process typically requires 12-15 years and extensive preclinical and clinical evaluations, with around 50 % of drug candidates failing due to unforeseen toxicity and inefficiencies.
Nanoparticle-driven 3D bioprinting improves efficiency by automating the creation of 3D cell cultures in 96-well plates within 10 minutes, reducing manual labor and enhancing model consistency. Technologies like NanoShuttle-PL further streamline the process by accelerating the formation of 3D structures, supporting rapid prototyping and scalability.
This technology also enables the production of hundreds or thousands of 3D structures in a single run, facilitating large-scale drug screening. It supports the construction of heterogeneous tissue models with multiple cell types, such as liver tissue constructs, which better mimic native tissue environments and improve predictions of drug responses, potentially reducing late-stage drug failures.3,4
Equipment Used in Nanoparticle-Driven 3D Bioprinting
Several advanced tools and platforms are employed in nanoparticle-driven 3D bioprinting to form 3D cell structures that enhance drug interaction studies.
The Greiner Bio-One NanoShuttle-PL is a prominent nanoparticle-based system that uses gold, iron oxide, and poly-L-lysine nanoparticles to label and aggregate cells into 3D spheroids via magnetic forces, facilitating rapid formation for high-throughput screening.1,5
Another example is the RASTRUM™ bioprinter, a high-throughput platform that employs drop-on-demand printing technology to deposit cells and matrix components into microplates, creating hydrogels that mimic tissue mechanics and allow for precise control of the cellular environment.6
Additionally, assay technologies like AlphaLISA and AlphaScreen offer high-sensitivity, no-wash protocols for detecting biomolecules in 3D cultures, providing crucial data on drug effects on cellular signaling and protein expression.7
These technologies collectively optimize the integration of 3D bioprinting into high-throughput screening workflows, minimizing manual intervention and boosting drug screening efficiency.8
Advancing Drug Efficacy with Realistic Models and Reduced Animal Testing
Nanoparticle-driven 3D bioprinting offers a more accurate in vitro model, enhancing preclinical studies by providing results that are more likely to translate to human patients. This enables the creation of complex tissue models, ranging from simple single-cell systems to sophisticated multi-cellular constructs, potentially replacing various stages of animal testing.
Recent studies by Hong et al. and Liu et al. demonstrate that 3D bioprinted models accurately replicate in vivo drug responses, including drug resistance and anti-tumor sensitivity. This improvement reduces the reliance on animal testing, which has about a 50 % failure rate in predicting human outcomes.9,10
The technology also enables the creation of personalized tissue models using patient-derived or genetically engineered cells, reducing the need for extensive animal testing by studying drug responses in individual genetic contexts.
Lastly, standardized 3D tissue constructs in multi-well plate formats facilitate high-throughput screening of large compound libraries, addressing ethical concerns and supporting biomedical research's 3Rs principle (Replacement, Reduction, and Refinement).8
Conclusion
Nanoparticle-driven 3D bioprinting represents a significant development in drug screening by creating more physiologically accurate tissue models, enhancing accuracy, efficiency, and ethical standards.
As this technology advances, it has the potential to revolutionize drug screening processes, ultimately leading to more efficient, cost-effective, and ethically sound approaches for introducing new therapies.
Revolutionizing drug discovery: High-throughput fragment screening with NexMR's X-Pulse benchtop NMR spectrometer
References and Further Reading
- Baillargeon, P., Shumate, J., Hou, S., Fernandez-Vega, V., Marques, N., Souza, G., Seldin, J., Spicer, TP., Scampavia, L. (2019). Automating a Magnetic 3D Spheroid Model Technology for High-Throughput Screening. SLAS technology, 24(4), 420–428. https://doi.org/10.1177/2472630319854337
- Revvity. (2024). Bioprinting workflow enables high-throughput drug discovery in 3D cell cultures. [Online] Revvity. Available at: https://www.revvity.com/blog/bioprinting-workflow-enables-high-throughput-drug-discovery-3d-cell-cultures?srsltid=AfmBOoqXwBQY_r_nAeyhf-pxpmS67DKIhRTzLZpCa6JZUn8bPNd6U3gg
- Mazzocchi, A., Soker, S., Skardal, A. (2019). 3D bioprinting for high-throughput screening: Drug screening, disease modeling, and precision medicine applications. Applied physics reviews. https://doi.org/10.1063/1.5056188
- Gao, G., Ahn, M., Cho, WW., Kim, BS., Cho, D. W. (2021). 3D Printing of Pharmaceutical Application: Drug Screening and Drug Delivery. Pharmaceutics. https://doi.org/10.3390/pharmaceutics13091373
- Greiner Bio-One. (2023). How is Magnetic 3D Bioprinting an Enabling Tool for High-Throughput Screening? [Online] Greiner Bio-One. Available at: https://focus.gbo.com/blog/3d/m3d-with-high-throughput-screening
- Inventia. (2024). RASTRUM™ Instrument. [Online] Inventia. Available at: https://inventia.life/platform
- Revvity. (2024). AlphaLISA and AlphaScreen No-wash Assays. [Online] Revvity. Available at: https://www.revvity.com/ask/alphalisa-and-alphascreen-no-wash-assays?srsltid=AfmBOor_W55wVcXMUzcGA_1MnZvcTe0PK_G8zZX68QincQtATUm8ItTN
- Engel, M., Belfiore, L., Aghaei, B., Sutija, M. (2022). Enabling high throughput drug discovery in 3D cell cultures through a novel bioprinting workflow. SLAS technology. https://doi.org/10.1016/j.slast.2021.10.002
- Hong S., Song JM. (2022). 3D bioprinted drug-resistant breast cancer spheroids for quantitative in situ evaluation of drug resistance. Acta Biomaterialia. https://doi.org/10.1016/j.actbio.2021.10.031
- Liu, Q., et al. (2023). 3D-bioprinted cholangiocarcinoma-on-a-chip model for evaluating drug responses. Bio-des. Manuf. https://doi.org/10.1007/s42242-022-00229-9
Disclaimer: The views expressed here are those of the author expressed in their private capacity and do not necessarily represent the views of AZoM.com Limited T/A AZoNetwork the owner and operator of this website. This disclaimer forms part of the Terms and conditions of use of this website.