A group of researchers reported in Nature Nanotechnology that raw copper (Cu) nanoparticles (NPs) with external surplus electrons maintain their non-oxidized condition after many months in the ambient atmosphere.
Study: Non-oxidized bare copper nanoparticles with surface excess electrons in air. Image Credit: Kateryna Kon/Shutterstock.com
Because of their advantages over their bulk form, copper nanoparticles have sparked much attention. Although spontaneous oxidation of Cu NPs can be reduced by coating the surfaces with various functionalities, preventing the production of non-oxidized raw Cu NPs in the air is still difficult.
The surface-accumulated surplus electrons encase Cu NPs produced on an electrode substrate with outstanding electron transport capacity, resulting in an extremely low work function.
The lack of Cu oxide constituents at the surface layer of bare Cu NPs is confirmed by atomic-scale chemical and structural investigations.
Conceptual energetics show that surface-accumulated excited electrons restrict oxygen binding and, as a result, prevent oxygen penetration into the Cu lattice, resulting in endothermic partial oxidation.
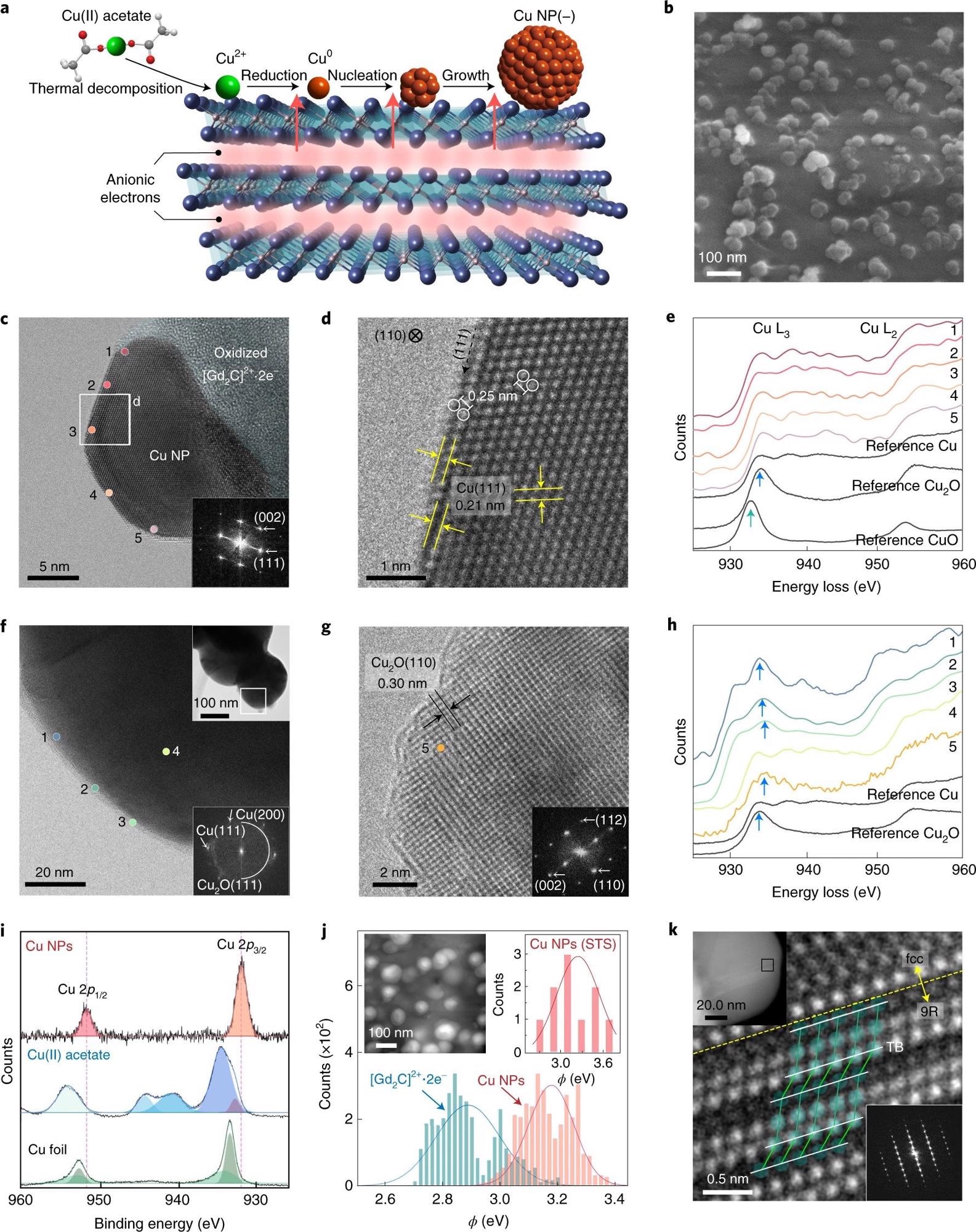
Figure 1. Negatively charged surface state of Cu NPs grown on a two-dimensional electride. a, Schematic of Cu NP growth on two-dimensional [Gd2C]2+·2e− electride. b, SEM image of Cu NPs on the electride. c,d, HR-TEM image of the as-prepared Cu NPs on the oxidized electride (inset: fast Fourier transform (FFT) pattern) (c) and enlarged TEM image showing the atomic structure of fcc Cu(110) (d). e, EELS data for positions 1–5 at the surface in c. f, HR-TEM image of conventional Cu NPs prepared by the thermal reduction method (top inset: overall morphology; bottom inset: FFT pattern). g. Enlarged TEM image of a conventional Cu NP, showing that the NP was oxidized to Cu2O with an interplanar distance of 0.30 nm. h, EELS data for positions 1–3 at the surface and position 4 in the bulk (as shown in f) and for position 5 (as shown in g). Except for position 4, all the spectra show the white lines of Cu2O (blue arrows). i, XPS spectra of Cu NPs, Cu(II) acetate and Cu foil. Two satellites (940.5 and 944.0 eV) for Cu(II) acetate are the characteristics of Cu2+. j, Work-function (ϕ) values of Cu NPs and [Gd2C]2+·2e− electride obtained by KPFM measurements. The peaks of the solid lines represent the average values (ϕav) for Cu NPs (~3.2 eV; orange) and [Gd2C]2+·2e− electride (~2.9 eV; blue) from all the measurements (solid bars). Inset: scanning tunnelling microscopy (STM) image of Cu NPs on [Gd2C]2+·2e− electride (left) and ϕav of Cu NPs obtained by STS (~3.3 eV) (the set-point conditions are as follows: bias voltage V = 3 V; tunnelling current I = 500 pA) (right). k, HR-TEM image of the 9R phase in Cu NPs from the boxed area in the top inset. The twin boundary (TB; white lines) and atomic structures (green balls) correspond to the 9R phase. Bottom inset: FFT pattern of the 9R phase. © Chung, K. et al. (2022).
Deterioration of Properties in Copper
Copper usually loses electrons, establishing a wide range of compounds, most of which have electronegativity of +1 and +2; these chemical states form exergonic cuprous oxide.
Cu's metallic characteristics decrease due to the development of Cu oxides in atmospheric environments, and the progression is expedited in Cu NPs with a high surface to volume ratio, which can be a cheaper substitute to valuable silver and gold equivalents in a variety of uses
Artificial Alteration of Copper Surface
The synthetic alteration of surface morphology via supplementary post-treatments is a required process in the fabrication of Cu NPs, including wet chemical methods and solid-state heating. Here, stabilizers are generally used to avoid particle agglomeration beyond nanometer scale.
Surface modification using thiolate compounds to prevent oxidation is one approach. The naturally generated Cu oxide compounds on the surfaces of Cu NPs may also be removed using a procedure based on chemical ablation, photoreduction, and thermal treatment.
While non-oxidized Cu NPs may be made by reducing Cu precursors in situ in the form of hybrids with an epoxy composite, there is no effective method to avoid bare Cu NPs from oxidizing in air.
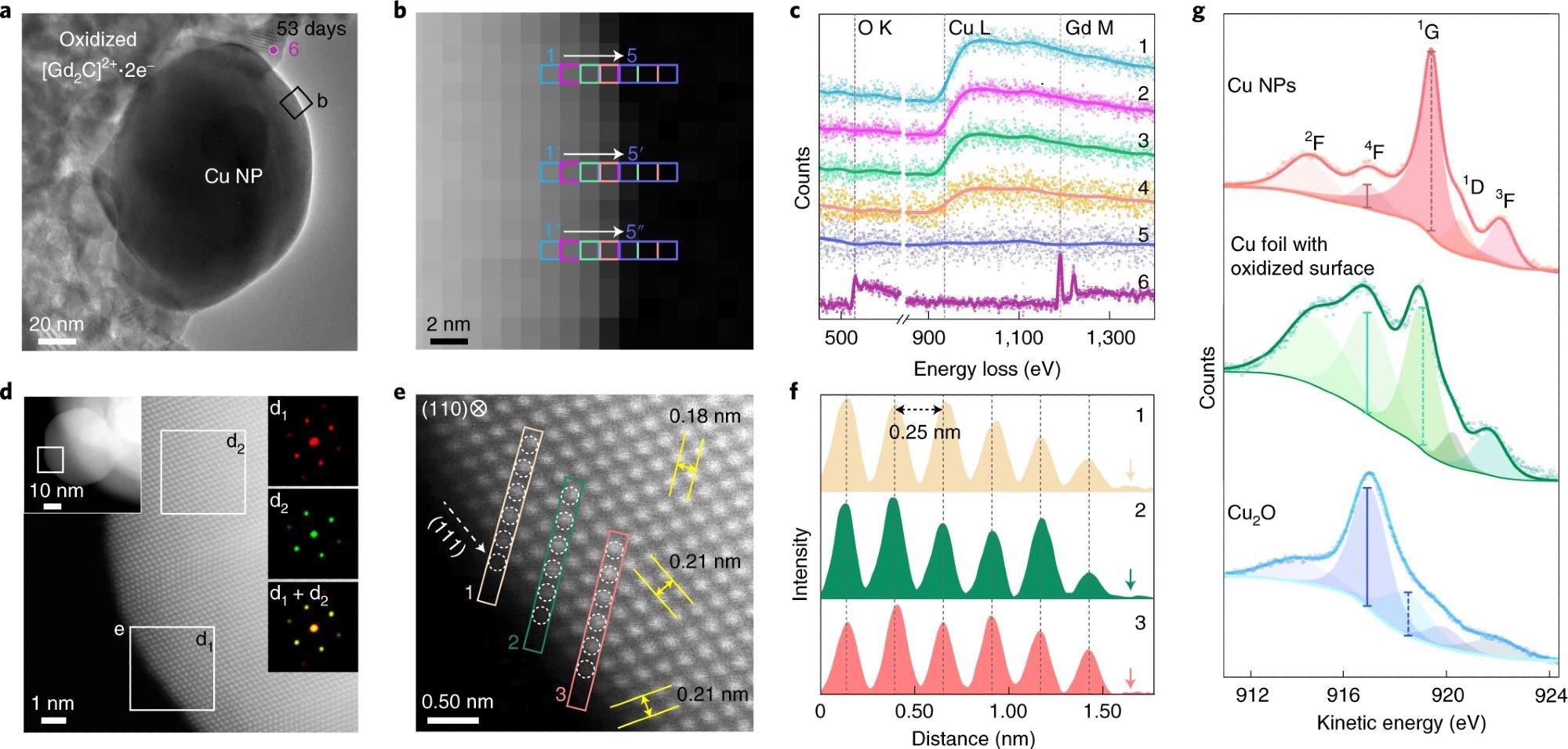
Figure 2. Surface analysis of air-exposed Cu NPs. a–c, EELS probing of the air-exposed Cu NP (53 days) shown in the TEM image (a), EELS mapping image with a nanometre-scale resolution through the acquisition of EELS signal from the three pixels marked as 1→5 (b) and the corresponding EELS data (c). Each spectrum is a sum of three different rows (n + n′ + n″). Spectrum 6 was obtained from oxidized [Gd2C]2+·2e− electride (marked as 6 in a). d, ADF STEM image of the boxed region in the left inset of the air-exposed Cu NP (right insets: FFT patterns of the boxed regions). e, Atomic arrangement at the surface region (boxed regions in d). Interplanar distances (yellow arrows) of 0.18 and 0.21 nm are of the (002) and (11) planes, respectively. f, Interatomic distance profiles of six atoms along with the boxed arrays (indicated as 1–3) in e up to the vacuum (arrows). g, Cu L3M45M45 AES data of the Cu NPs (top), Cu foil with the oxidized surface of 1 nm thickness (middle) and Cu2O powder (bottom). Cu L3M45M45 AES data are fitted into five peaks. For Cu NPs, the kinetic energies and peak-area values for Cu NPs are consistent with a previous report35. The strong peak at 916.8 eV of Cu foil comes from the surface oxide, as revealed by the XPS data (Fig. 1i). In the Cu2O spectrum, the peak intensity at 916.8 eV is the strongest. Different intensity ratios between the peak at 916.8 eV (solid vertical line) and that between 918 and 919.2 eV (dashed vertical lines) for Cu NP with Cu foil indicate that 1-nm-thick surface oxide generates large discrepancy in the AES data, implying the non-oxidized surface state of Cu NPs. © Chung, K. et al. (2022).
Classic Cathodic Protection Method
Because of the planar polarity among metal cations and O2 anions, metal oxides develop at the surface. As a result of this electrochemical reaction, it is possible to avoid metal from oxidizing by limiting the escape of electrons from copper atoms or by inhibiting the binding of oxygen.
Limitations of Cathodic Protection for NPs
Due to the impracticality of directing each NP to provide electrons the cathodic protection approach for metal NPs is challenging to employ under ambient settings.
To synthesize bare Cu NPs with additional negatively charged ions, the researchers devised a coherent one-step fabrication process that allows for continuous development and transfer of electrons, in which Cu NPs are produced on the surface of an electron-donating scaffold.
Using artificial electrodes as electron-donating materials, they effectively exhibit non-oxidized Cu NPs in the air for many months, even on the atomic scale. There were no further post-treatments or surface modification procedures needed, which was a massive plus.
Utilization of Electrides as Support for Protection in NPs
Electrides are electric crystals with unusual features based on surplus anionic electrons filling a morphological area of 0.4nm, which were being employed as a support in the current production of bare Cu NPs by researchers.
The primary distinguishing feature of electrides is their low work function, which enables anionic electrons to be effectively transferred to a material with a greater work function.
Anionic electrons have shown to be physically and chemically bonded materials including organometallic nanoparticles and carbon nanofibers, resulting in improved catalysis and dispersion capabilities.
The researchers were able to create bare Cu NPs with extra electrons collected on the surface, resulting in ultrahigh chemical inertness under ambient environments.
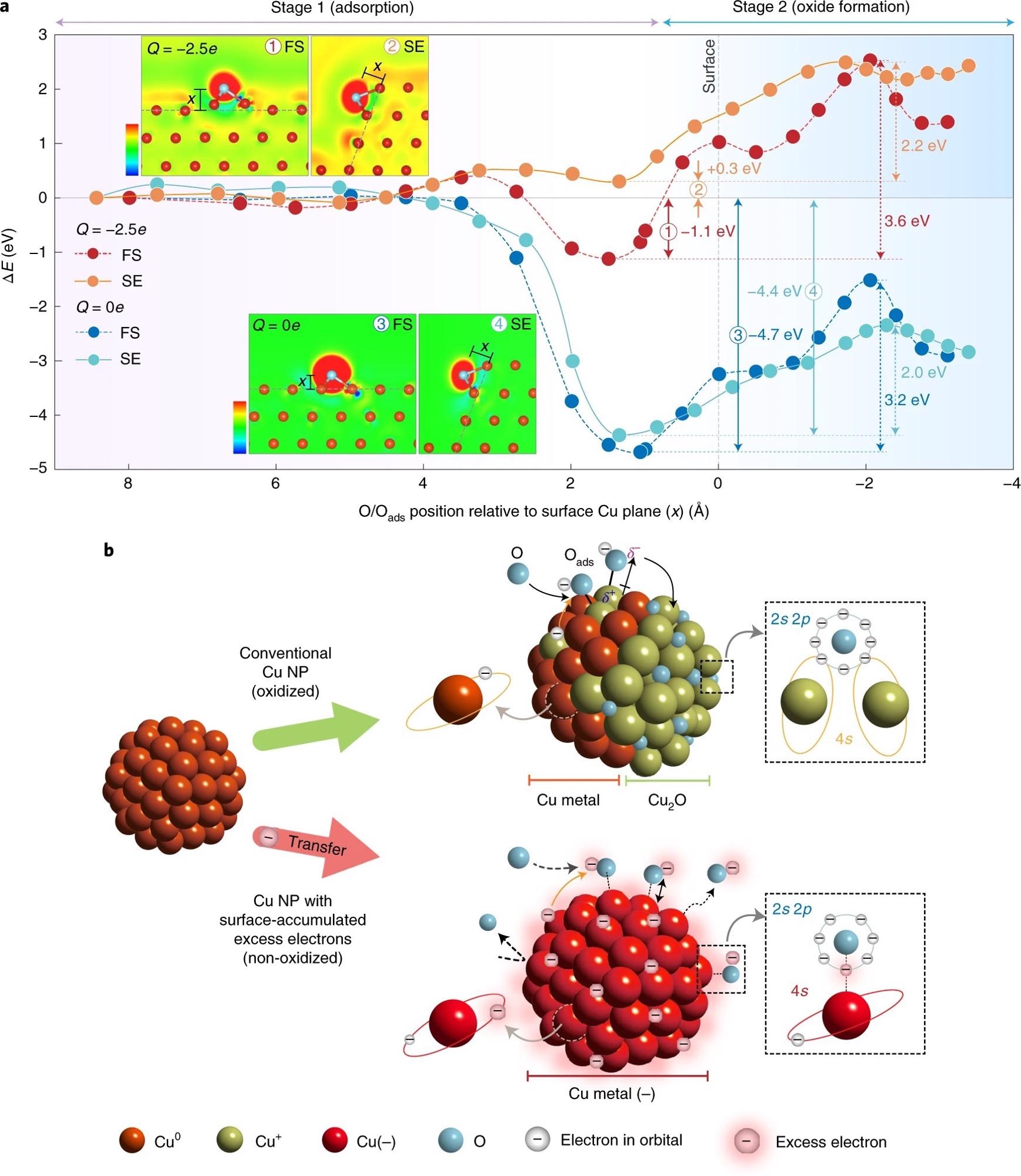
Figure 3. Theoretical analysis and model of oxidation-resistant Cu NPs. a, Relative energy profiles of the adsorption of O atom onto neutral (Q = 0e) (blue circle, FS; cyan circle, SE) and charged (Q = −2.5e) fcc Cu(111) facets (red circle, FS; orange circle, SE). The insets show the plots of excess electron density for O-adsorbed FS and SE of Cu(111) facets in neutral (Q = 0e) and charged (Q = −2.5e) states. The blue (red) colour represents the region of electron depletion (augmentation) with reference to neutral systems (scale bars represent the range from −0.037 to 0.037e Å–3 and from −0.057 to 0.057e Å–3 for FS and SE of Cu, respectively). b, Schematic of the model for the oxidation process of conventional Cu NPs (top) and non-oxidized Cu NPs with surface-accumulated excess electrons (bottom). δ+ and δ– correspond to the amount of electron loss in Cu and electron gain in O, respectively. © Chung, K. et al. (2022).
Research Findings and Conclusion
A highly reactive surface state mimicking typical cathodic protection was used to manufacture non-oxidized bare Cu NPs in the air by researchers. The surface crystal lattice of FCC Cu in air-exposed Cu NPs after 15 and 30 days in the air confirms the transfer of excess electrons to Cu NPs.
After 1 hour in distilled water, mass-produced raw Cu NPs showed no surface oxidation, indicating superior corrosion resistance. The increased performance of non-oxidized Cu NPs in catalytic processes and electrodes is supported by the excess electrons communicated from the electrides.
Less expensive metal nanoparticles may now replace noble gold and silver nanoparticles in a broad variety of applications. A hybrid complex of metal NPs and electrides is particularly useful for catalytic processes requiring efficient electron transport.
Reference
Chung, K. et al. (2022). Non-oxidized bare copper nanoparticles with surface excess electrons in air. Nature Nanotechnology. Available at: https://www.nature.com/articles/s41565-021-01070-4
Disclaimer: The views expressed here are those of the author expressed in their private capacity and do not necessarily represent the views of AZoM.com Limited T/A AZoNetwork the owner and operator of this website. This disclaimer forms part of the Terms and conditions of use of this website.