Nanoparticles are objects with a diameter ranging from 1 to 100 nm.1 They have a myriad of applications in medical sciences, photonics, chemical synthesis, and catalysis.2-5
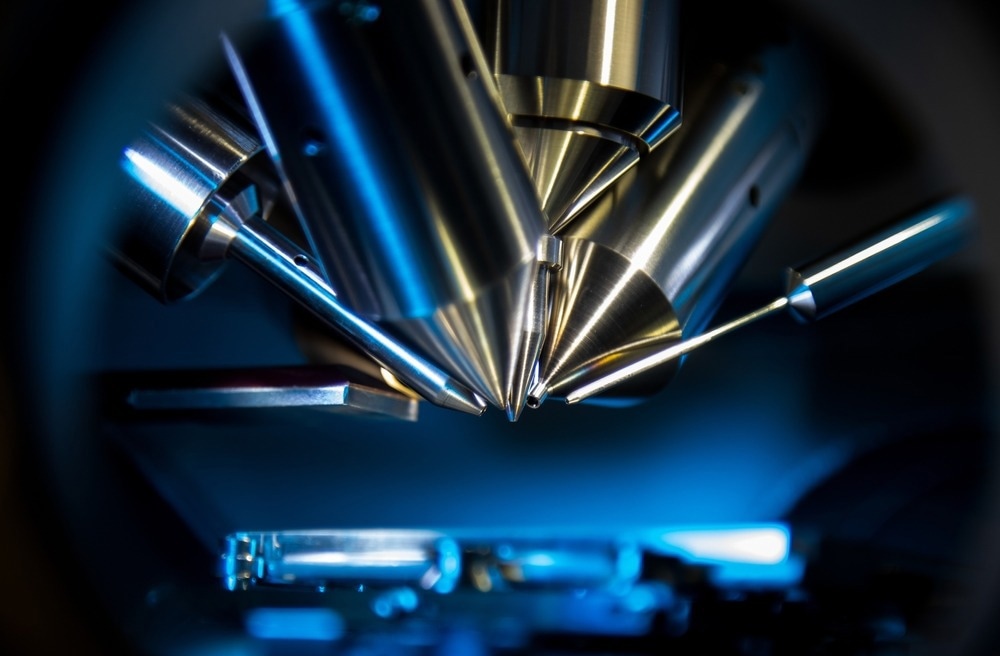
Image Credit: Intothelight Photography/Shutterstockcom
A crucial element in both the synthesis and functionality of nanoparticles involves the attachment of ligands.6 The appropriate choice of ligands can enhance the solubility of nanoparticles or affect their aggregation and self-assembly behavior.7 Ligands can also modify properties such as optical absorption or emission.
The overall properties of nanoparticles depend on the material they are made from, their size, shape, and any attached ligands. Consequently, nanoparticle detection methods must be sensitive to several or all of these parameters.
Types of Nanoparticle Detection Techniques
There are various classes of nanoparticle detection methods, including optical detection, electron microscopy, mass spectrometry, dynamic light scattering (DLS), and field-flow fractionation (FFF).
Optical Detection
Optical imaging of nanoparticles with visible light can be challenging because the diffraction limit of light is larger than that of many nanoparticles, which is why electron microscopy is often favored.
However, optical detection techniques like absorption, fluorescence, and surface plasmon resonant (SPR) methods can still provide indirect size information and be valuable tools for profiling the optical properties of nanoparticles.
Absorption spectroscopy measures how much light a sample absorbs, typically as a function of excitation wavelength. Many nanoparticles, particularly those made from gold, have high visible absorption cross-sections, making them useful in enhancing the light-collection efficiencies of devices such as solar cells.8
The optical absorption profile of a nanoparticle is often size-dependent, with plasmon peak resonances often shifting to longer wavelengths as the nanoparticle size increases.9
Optical absorption measurements are straightforward to perform and can rapidly provide size profiles in well-characterized systems. Emission or fluorescence properties can provide similar information but can also measure nanoparticle concentrations.10
Many nanoparticles are used to generate surface plasmon resonance applications, such as cell detection or biomolecular sensing.11 However, SPR can also be used to detect nanoparticles themselves.
SPR is often used as a highly sensitive probe for the binding of nanoparticles or another species to a surface, with signal shifts or enhancements depending on the exact geometry of the surface binding and interaction.
Electron Microscopy
Both transmission electron microscopy (TEM) and scanning electron microscopy (SEM) are widely used for nanoparticle characterization. These techniques possess some of the best spatial resolutions among imaging techniques, making them exceptionally suited to delineating the shape and sizes of nanoparticles.12
Another advantage of electron microscopies is that the high-energy electrons used to image a sample can also induce the emission of X-Rays from the sample. These X-Rays have energies specific to different elements and species, allowing for the simultaneous characterization of the particle's elemental composition.
As well as for final product characterization, some electron microscopy methods can be used to monitor assembly or growth processes.5 Understanding how nanoparticles self-assemble provides valuable insights for researchers designing nanoparticles with specific geometries or sizes.
Mass Spectrometry
Mass spectrometry is a useful tool in nanoparticle detection, aiding in the identification of a sample’s elemental composition. It is also used to understand the repeating units that comprise a nanoparticle and their relative stability.13
This technique separates species based on their mass-to-charge ratio and analyzes their fragmentation patterns. Since a nanoparticle sample typically exhibits a size distribution, mass spectrometry can determine which particle types are most abundant, often correlating directly with the energetic stability of those nanoparticles. Energetically stable species are more likely to form as they represent the most stable configurations.
Dynamic Light Scattering
DLS is a widely available technique with numerous commercial solutions. It is used to evaluate the particle size distribution and sizing within a sample and offers excellent spatial resolution.
DLS uses optical measurements of scattered light to determine a sample's diffusion coefficient, which correlates to the particle radii.
While DLS is not a direct imaging method, it is one of the fastest and easiest methods for estimating particle sizes, especially in dilute solutions. Measurements of zeta potentials can also be used to evaluate particle stability.
However, DLS has limitations in concentrated solutions or when the scattering contrast is poor.
Field-Flow Fractionation
FFF works by separating particles based on their sizes and physical properties.
The sample is passed through a thin, flowing channel where an external field is applied. The time it takes for particles to travel through the channel depends on their interaction strength with the field and their size, making FFF particularly effective for separating high-mass particles and characterizing their charge properties using the external fields.
Challenges and Considerations
The size of nanoparticles inherently complicates their measurement in direct imaging experiments. However, numerous indirect techniques, such as optical absorption and DLS, often provide sufficient information for assessing particle sizing.
In solutions with high nanoparticle concentrations, measurement can be particularly problematic due to significant light scattering, which poses challenges for optical methods.
However, these techniques have commercially available solutions, and many of the required analytical methods are also automated.
Often, multiple techniques can be combined to provide complementary information, offering a more comprehensive understanding of the nanoparticle’s composition and structure when required.
More from AZoNano: Emerging Electron Microscopy Techniques for Quantum Research
References and Further Reading
- Sardar, R., Funston, AM., Mulvaney, P., Murray, RW. (2009). Gold nanoparticles: Past, present, and future. Langmuir. doi.org/10.1021/la9019475
- Huang, X., El-Sayed, MA. (2010). Gold nanoparticles: Optical properties and implementations in cancer diagnosis and photothermal therapy. Journal of Advanced Research. doi.org/10.1016/j.jare.2010.02.002
- Hertzog, M., Wang, M., Mony, J., Börjesson, K. (2019). Strong light-matter interactions: A new direction within chemistry. Chemical Society Reviews. doi.org/10.1039/c8cs00193f
- Schmermund, L., et al. (2019). Photo-Biocatalysis : Biotransformations in the Presence of Light. ACS Catalysis. doi.org/10.1021/acscatal.9b00656
- Grzelak, D., Szustakiewicz, P., Tollan, C., Raj, S., Král, P., Lewandowski, W., Liz-Marzán, L. M. (2020). In Situ Tracking of Colloidally Stable and Ordered Assemblies of Gold Nanorods. Journal of the American Chemical Society. doi.org/10.1021/jacs.0c06446
- Heuer-Jungemann, A., et al. (2019). The role of ligands in the chemical synthesis and applications of inorganic nanoparticles. Chemical Reviews. doi.org/10.1021/acs.chemrev.8b00733
- Sanità, G., Carrese, B., Lamberti, A. (2020). Nanoparticle Surface Functionalization: How to Improve Biocompatibility and Cellular Internalization. Frontiers in Molecular Biosciences. doi.org/10.3389/fmolb.2020.587012
- Notarianni, M., Vernon, K., Chou, A., Aljada, M., Liu, J., Motta, N. (2014). Plasmonic effect of gold nanoparticles in organic solar cells. Solar Energy. doi.org/10.1016/j.solener.2013.09.026
- Balamurugan, B., Maruyama, T. (2005). Evidence of an enhanced interband absorption in Au nanoparticles: Size-dependent electronic structure and optical properties. Applied Physics Letters. doi.org/10.1063/1.2077834
- Jamil, S., Liaqat, U., Ahmed, N., Ahmed, R., Umar, ZA., Baig, MA. (2022). The role of nanoparticles concentration in the emission intensity enhancement of the laser-produced aluminum plasma. Physica B: Condensed Matter. doi.org/10.1016/j.physb.2021.413620
- Nguyen, HH., Park, J., Kang, S., Kim, M. (2015). Surface plasmon resonance: A versatile technique for biosensor applications. Sensors. doi.org/10.3390/s150510481
- Wang, ZL. (2003). New Developments in Transmission Electron Microscopy for Nanotechnology. Advanced Materials. doi.org/10.1002/adma.200300384
- Kasuya, A., et al. (2004). Ultra-stable nanoparticles of CdSe revealed from mass spectrometry. Nature Materials Letters. doi.org/10.1038/nmat1056
Disclaimer: The views expressed here are those of the author expressed in their private capacity and do not necessarily represent the views of AZoM.com Limited T/A AZoNetwork the owner and operator of this website. This disclaimer forms part of the Terms and conditions of use of this website.