It seems like the stuff of science fiction movies – a cloaking device to make objects invisible, a super lens that can see sharp details far below the wavelength of light. Those are the glamour concepts that are driving the new field of metamaterials research, but a team of experts led by Penn State is hoping to go beyond the glamour to make metamaterials a part of our everyday lives. Directed by electrical engineer Doug Werner, an authority on antenna design, the Penn State team is partnering with Osaka University in Japan, University of Birmingham UK, University of Puerto Rico, as well as Purdue and the University of Pennsylvania to develop metamaterials devices to address major societal issues in energy, transportation, health, and telecommunications. Center For Metamaterials Study Earlier this year, Werner recruited a large, multitalented group of researchers to create a center for metamaterials study at Penn State, with the goal of competing for a National Science Foundation Engineering Research Center (ERC). The NSF funds ERCs to undertake long-term, high value research intended to provide significant benefits to society. With the support of his colleagues at Penn State and partner universities, Werner has begun the highly competitive ERC process. Metamaterials "Basically a metamaterial is an artificially engineered material that has highly desirable properties that don't occur in nature," Werner explains as he calls up images of left-hand refracted light waves on his computer screen. "If they did they would be very useful in a lot of devices and applications. But we can take combinations of materials that do occur in nature and put them together, and they will act in such a way that the overall response of that material is something different than the response of the parts. We can use that idea to create new materials. It's an extremely exciting area in which we're exploring all these materials and properties." Computational Modelling Tools Theresa MayerWerner develops new metamaterials using a set of computational modeling tools to predict electromagnetic behavior. Inspired by nature, these relatively new global optimization techniques, called genetic algorithms and particle swarm, are widely used by engineers and scientists, but Werner has tailored the techniques to his own purpose. "There are a lot of other groups around the country looking at different metamaterials for different applications. But I would say one of the things that differentiates our group from the others is that we're actually going beyond just looking at different kinds of metamaterials and analyzing their properties; we're actually trying to optimize the underlying structure and the materials used to make the metamaterial." The two computational methods are very powerful, according to Werner. Genetic algorithms are based on the Darwinian notion of evolution through natural selection. With GA, he encodes the basic design of a material with its characteristic properties into the equivalent of a chromosome. A number of chromosomes are randomly selected to create a population, which is ranked according to how well they fit a predetermined metamaterials design. The worst performers are weeded out and new chromosomes added in a process called crossover. Parts of the genetic material from the better performers are put together to make up a new generation, with random mutations added to shake up the process. Doing this for successive generations eventually evolves the optimal design through crossover, mutation, and natural selection. Biology Based Computation The other computational method is also biology based. Particle swarm optimization technique was inspired by the behavior of flocks of birds and swarms of bees. As bees search for the best source of pollen, they communicate their finds with others in the swarm. The bees keep track of their best local find and also of the best finds within the swarm. This has been applied to the finding of the metamaterials with the best properties. "Using nature inspired methods has been one of the themes or our work," Werner says. Most recently, Werner's research group has been applying these nature inspired optimization techniques to develop metamaterial coatings that have application in the design of a narrowband tunable "electromagnetic cloak of invisibility." Making Metamaterials Once an optimal metamaterial structure has been found, the job of actually making it is turned over to the Theresa Mayer group. Mayer, an electrical engineer, is deputy director and chief scientist for the metamaterials ERC. Her group uses contact lithography, a high throughput technique, to fabricate the larger, simpler structures. For complex structures at the micro or nanoscale, they employ ebeam lithography, which uses a stream of electrons to carve 3-D patterns. Their early metamaterial geometries when pixilized for analysis looked like a Scrabble board covered by random patterns of metallic and nonmetallic tiles. These structures proved to be too small and complex for Mayer's group to fabricate. "We had to include a rule set in the genetic algorithms that would exclude single pixels or any pixels that are diagonally connected," Werner says, as more organized images appear on the screen. "You can see that the structures are much simpler. We've done some now on the order of 3 microns. These are the kinds of complex patterns we are now routinely designing in our group and fabricating in Theresa's group." 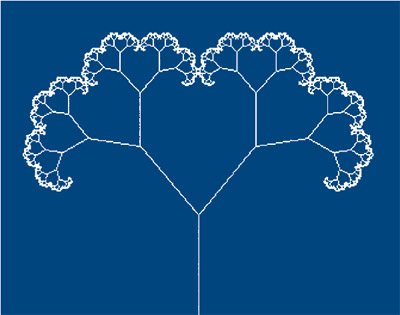 Figure 1. Fractals occur in nature – trees, branches, river basins, and snowflakes for example. They can also be used to design metamaterials. Metamaterial Applications Metamaterials have already found their way into commercial products. In micro and millimeter wave, they are already being used to make better antennas. Toyota is developing metamaterials for automotive applications. Werner, Mayer, and chemist Tom Mallouk are looking at a radically different way of designing solar cells using nanowire antennas that are half the wavelength of light. The resonance properties of the nanowires could make them potentially far more efficient than current solar cells. "It's real engineering of materials," says Werner. "Because of this capability to design and fabricate new materials, a lot of companies are talking to us about using these metamaterials for various projects of interest to them. Then, of course, this ERC is focused on these metamaterials, but mainly on the next generation and beyond of metamaterials. There is a lot of good science and good engineering that is yet to be done. A center like that would push the technology forward in a big way." Ghost in the Machine Elena Semouchkina of the Materials Research Institute studies the physics of the electromagnetic interactions between materials. EM waves propagate through a material according to principles laid out by Maxwell. Using a powerful modeling formula devised by Penn State professor of electrical engineering Raj Mittra to solve Maxwell's Equations for metamaterials, Semouchkina analyzes the electromagnetic wave model as it propagates through the simulated metamaterial structures on her computer screen, even running backwards in the case of negative index materials. She and her husband, visiting scientist George Semouchkin, are also collaborators in Werner's ERC team. With advanced degrees in physics and electrical engineering from Russia, and a doctorate in materials from Penn State, Elena Semouchkina will be a key theoretician in a number of metamaterials projects involving terahertz and radio frequency devices. Instead of the classical cut wires and split ring resonators used by Pendry and Smith, Semouchkina studies high permittivity cylinders embedded into a low permittivity matrix, a process developed in Penn State's Center for Dielectric Studies. The cylinders are nonmetallic resonators linked together in a periodic structure. They can be designed to perform at microwave, millimeter wave, and terahertz frequencies. Resonance Effects In Metamaterials "We study resonance effects in metamaterials," Semouchkina explains. "If you are marching in unison across a bridge, the resonance effect enhances the power of the vibration. Similarly, electromagnetic resonators enhance EM power transmission in a metamaterial. With many resonators, the field is enhanced further. We use small particles that are too small to be noticed by the wave because they are smaller than the wavelength, and observe an enhancement effect within resonators that is called coupling. We have observed some effects that have not been noticed or taken into account before. "A broad group of new architectures can now be based on this concept, in which dielectric resonances in periodic structures are tailored for novel microwave and mm-wave applications, such as antennas with beam steering, filter devices, multiplexers, phase shifters or electro-optical modulators," Semouchkina remarks. Dielectric Structures Of particular interest are dielectric structures based on fractals, patterns of similar shapes repeated in decreasing size that can be found in many natural phenomena. Examples of fractals found in nature include lightning, snowflakes, river basins and tree branches. Chaos science uses fractal geometry to model complex data, such as weather. In the last 10 years, fractal designs have been used to create miniature multiband antennas and to reduce the size of stored computer images. Semouchkina models complex fractals which are then fabricated by her collaborator Prof. Miyamoto of Osaka University, Japan. In a development with interesting possibilities for energy harvesting, Professor Miyamoto has reported the containment of electromagnetic energy in an elaborate fractal called a Menger Sponge (see page 4.) The concept of a dielectric Menger Sponge, which looks like a Rubik's cube with increasingly smaller square holes drilled in each face, was used in a 2006 Taiwanese science fiction film as a device to capture ghosts. The film, called Silk in English, was very loosely based on Prof. Miyamoto's work, but he doubts his machine is capable of capturing spirits in its metamaterial architecture. Metamaterials - Designs for Living Spaces "The field of metamaterials can be related to a biological organism, such as the human body," says Penn State mechanical engineer Akhlesh Lakhtakia, the ERC's designated theorist. A polymath with wide-ranging interests and considerable expertise in many areas, from black holes to nanotechnology, children's literature to cricket, Lakhtakia likes to think in large terms. Along with his colleague Tom Mackay, a mathematician at Edinburgh University, Lakhtakia has published some widely discussed papers on metamaterials, one predicting that negative refraction in vacuum could make the exact location of stellar objects unknowable, and another proposing a relatively simple method for making superlenses by blending a metallic material with a magnetic material into spheres about the size of the wavelength of light. Lakhtakia is also noted for his development of sculptured thin films as an optical material. Metamaterials Vision A year ago, Lakhtakia received a call from Mike Lanagan, associate director of the Materials Research Institute, asking him to speak to MRI faculty on the topic of metamaterials. That talk, he says, started him thinking about the larger subject of metamaterials research. A short time later he was asked for an article on the same topic for Optics and Photonics News, which led him further along the path toward a grand vision for metamaterials. By the time the ERC group was forming, he had developed a framework for a possible metamaterials roadmap. In April 2007, in a teleconference call between researchers involved in the ERC proposal, the collaborators struggled to come up with a vision statement, one of the proposal's requirements. It was clear that cloaking devices and perfect lenses, though exciting to the public, would be of limited benefit to society. A big vision was needed, such as the one proposed by physicist Richard Feynman in regards to the promise of nanotechnology or Norbert Wiener's in the early days of computing and information technology. After most of an afternoon spent discussing the required vision statement, the teleconference was breaking up without coming to any clear resolution. Professor Werner asked for final comments. Silent until that moment, Lakhtakia responded by telephone from his office across campus. Using a metaphor drawn from biology, he outlined a vision of metamaterials as cells within a living organism with specific functions based on their structure - cells for communication, for energy harvesting, for structural integrity, and so forth. Simple metamaterial devices, some with periodic structures, some aperiodic, and some combining both, would be assembled into what he called a Supercell, a collection of devices designed to perform complex functions, such as controlling the energy needs of a living space or office building. Supercells, he said, are reconfigurable and multifunctional. "The ultimate aim of this theory is to think of living spaces as complex inanimate organisms capable of interacting with us and with each other." Nanotechnology is the key that will allow devices to shrink from the macro to the micro and nanoscale, making structured materials adaptable and reconfigurable, according to Lakhtakia. "The key is to exploit nanotechnology for cellularity and multifunctionality - cellularity at the structural level and multifunctionality within systems." New Capabilities Through Metamaterials Vistas of rationally designed land and cityscapes appeared as he described his vision of the metamaterials future. "Some of these theories may not happen, but some will. Call it informed vision," he said, "because we are developing new capabilities through nanotechnology. There is a parallel with the human genome project. We now have the capability of determining the root causes for biological problems. But the project itself did not determine the causes or cure the diseases. That was all left for further research. But now we have a book. This is a vision in that sense." Metamaterials - A Tree With Many Branches Theresa Mayer took Lakhtakia's roadmap and within a few days had crafted it into a scenario for the ERC vision statement, which was due by the end of the week. The ERC proposal was now focused on three metamaterials device test beds that would combine Semouchkina's physics, Werner's powerful algorithm designs, and Mayer's nano and microfabrication skills, along with the contributions of more than 20 collaborators in the U.S and abroad, each of them well-known in his or her field. In addition to the group of university researchers, Werner and Lanagan have recruited the support of major corporations and small and mid-sized companies interested in helping to commercialize the metamaterials devices they expected would come out of their test beds. 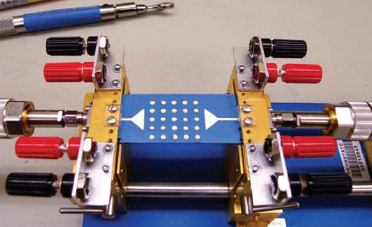 Figure 2. Resonant metamaterial – high permittivity dielectric resonators embedded in a low permittivity substrate for microwave transmission. Using ceramics instead of metals allows these devices to be scaled up to higher frequencies with low loss. The outcome of the ERC proposal is still many months in the future. In the meantime, the metamaterials initiative will continue, with support from the Materials Research Institute and the University. The opportunities are too large to let the team fall apart. Generation of Electromagnetic Energy The vision is that the control, harvesting, and generation of electromagnetic energy through structured manmade materials will one day lead to a better world - more energy with less pollution, better healthcare, greater privacy and security, safer transportation, a stronger economy. Those at least, are the hopes and visions of the new field of metamaterials. At the moment these dreams are like the fractal design of an oak tree that lies within an acorn. But with proper care, who knows how big this metamaterials tree can grow. |