Nanowires have piqued the interest of nanotechnology enthusiasts due to their applications in electronic and optoelectronic devices, and they are expected to play a significant role in future device technology.
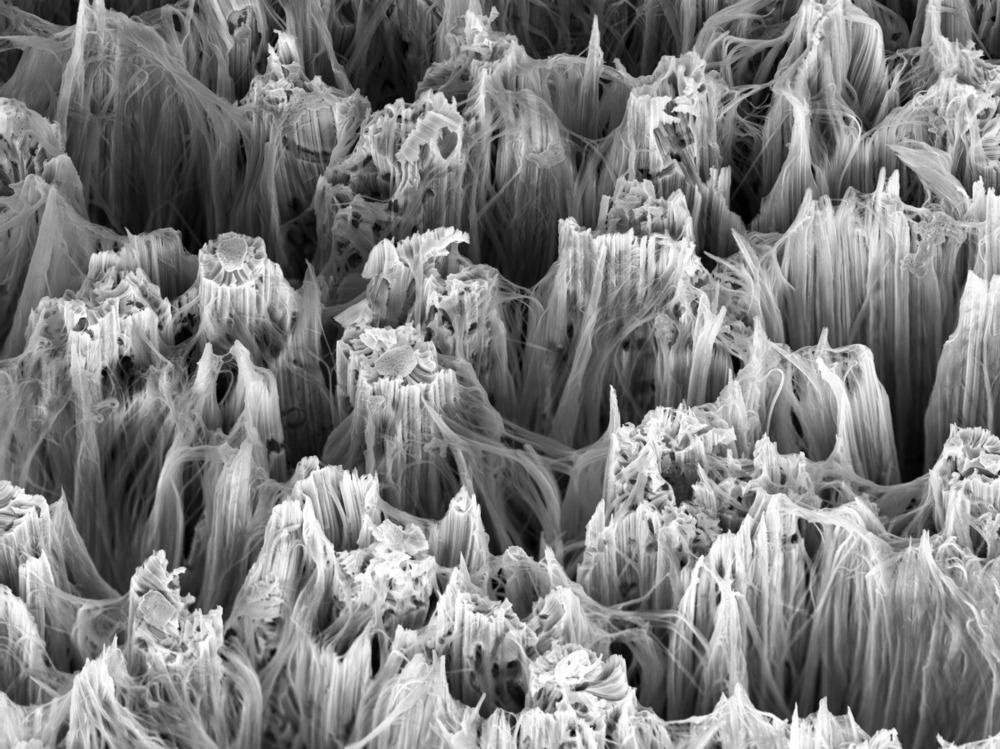
Image Credit: Roberto Lo Savio/Shutterstock.com
Many room-temperature applications have already been demonstrated, including field-effect transistors, solar cells, biosensors, and many others. This article aims to provide an overview of major nanowire synthesis techniques as well as recent developments in industrial applications.
Nanowires And Their Properties
Nanowires (NWs) are 1D nanostructures in the shape of wires with diameters of a few tens of nanometers or less and lengths ranging from a few micrometers to centimeters. As quantum mechanical effects are significant at nanoscales, such wires are also referred to as "quantum wires." There are many different types of nanowires, including semiconducting (Si, GaN, InP, etc.), metallic (Au, Pt, Ni), insulating (SiO2, TiO2), conductive polymers (polypyrrole, PANI), and molecular nanowires, which are made up of repeated organic (DNA) or inorganic molecular units.
Nanowires have a high surface-to-volume ratio and a low defect density, making them very sensitive to surface chemistry changes for sensing and biomedical applications. The optical and thermal properties of nanowires can be tuned by varying their diameter.
With their unique one-dimensional structure and remarkable optical properties, nanowires also open up new possibilities for realizing high-efficiency optoelectronics devices. Nanowires are ideal components for the bio-detection of pathogens and biological and chemical species due to their small size. These inherent properties make them a better candidate for their wide range of applications.
The synthesis method precisely determines nanowire growth and dimension at the atomic level. Nanowires can be produced using either a top-down or bottom-up approach. In a top-down approach, bulk materials are reduced to small portions by lithography or milling, whereas fundamental atoms are combined to form nanowires in bottom-up methods using the vapor-liquid-solid method.
Top-Down Method
Lithography
Electron beam lithography, widely used in the microelectronics industry, is one of the most popular top-down techniques for nanowire fabrication. This method entails the deposition of a resist material, such as PMMA, that acts like photographic film. A focused beam of high-energy electrons (typically > 30 kV) is scanned across the surface, tracing out the desired pattern. To make vertical nanowires, a hole pattern will be etched into the surface of the target material, while etching lines will make horizontal nanowires into the target material's surface.
Scanning probe lithography (SPL) is a group of nanolithography methods that use scanning probes to pattern material at the nanoscale. It is a mask-less approach and can achieve resolutions of less than 10 nm.
Chemical Etching
Chemical etching is a direct method for producing high-quality, well-aligned nanowire arrays with large-area homogeneity in a short amount of time. For example, for the synthesis of Si nanowires, a clean Silicon wafer is electroplated with Ag nanoparticles in this technique by immersing it in a solution containing HF and AgNO3, followed by etching in HF and H2O2.
After that, the wafer is immersed in dilute HNO3 to remove the Ag catalyst and produce a uniform array of Si nanowires. Finally, by scratching them off the wafer, free-standing individual nanowires can be obtained. The distribution of the patterned metal nanoparticles on the silicon surface determines the density and size of the as-prepared Si nanowires.
Bottom-Up Method
Vapor-Liquid-Solid method (VLS).
The vapor-liquid-solid method is a common method for producing nanowires (VLS). This method, for example, uses source material from a feed gas, such as silane, for the synthesis of Si nanowires. A catalyst, such as gold nanocluster, is deposited on a substrate before being transferred to a chemical vapor deposition tube furnace. The source gas enters and begins to saturate these nanoclusters. When the source reaches supersaturation, it solidifies and expands outward from the nanocluster. By simply turning off the source, the final length of the nanowire can be adjusted.
VSS (vapor-solid-solid) synthesis of nanowires (NWs) is similar to VLS. It begins with the thermolytic decomposition of a silicon precursor (typically phenylsilane). Unlike VLS, the catalytic seed remains solid when the substrate is annealed at high temperatures. This type of synthesis is commonly used to create germanide or silicide nanowires.
Solution-Phase Synthesis
The term "solution-phase synthesis" refers to techniques for growing nanowires in solution. Compared to other methods, they can produce nanowires of many different materials in very large quantities. This method is especially useful for creating gold, lead, platinum, and silver nanowires. Researchers, for example, used solution-phase synthesis to produce single-crystal Ge nanowires. At 275°C, a mixture of GeCl4, phenyl-GeCl3, and dry hexane is reduced by metallic Na dispersion in toluene. This method produced nanowires with diameters ranging from 7 to 30 nm and lengths of up to 10 m. By using an excess of Na in the reaction, the wire diameter could be reduced to 5 nm.
Non-Catalytic Growth
Nanowires can also be grown without catalysts, giving them the benefit of purity while also reducing the number of technological steps required to produce them. For example, researchers have reported synthesizing copper oxide nanowires without catalyst by heating Cu foils in the air. The annealing temperature and growth time are discovered to play an important role in the morphology of CuO nanowires, such as the length and diameter.
Industrial Applications of Nanowires
Scientists reported a solar cell out of graphene-coated with zinc oxide nanowires and cadmium sulfide nanowires. The researchers believe that this method will enable the production of low-cost, flexible solar cells with competitive efficiency. Using nanowire electrodes, flat panel displays could be thinner and more flexible than current flat panel displays.
Manganese dioxide nanowires are being used to create flexible capacitors. The capacitors will be embedded in the fabric to provide energy storage for wearable electronics.
Scientists used an electrified filter made of carbon nanotubes and silver nanowires to kill bacteria in water. In healthcare applications, researchers are working on a nanowire-based sensor to detect indicators of bladder and prostate cancer in urine samples.
Nanowires and nanowire synthesis at Joanneum Research Materials
Nanowires and nanowire synthesis at Joanneum Research Material. Video Credit: SUSTAINair H2020 Project/YouTube.com
Comparison of the Fabrication Approach
There are several key differences between the two methods for creating nanowires. The lithography process produces well-ordered structures with precise distances and positions. Furthermore, no metal catalyst is used in the fabrication of the NW. The main disadvantage of the lithographic technique is that the resolution of photolithography is limited by the wavelength of light used, and it is frequently not practical for small nanowires. Furthermore, much more independent parameters are involved in lithography, resulting in a longer and more complicated process.
Bottom-up approaches had the advantage of having a high NW density, a long NW length, and a homogeneous distribution over a large area. The growth takes place in extremely clean and reproducible conditions. However, it has some drawbacks. Because the VLS and VSS growth rely on a metal catalyst, metal contamination is one of the major drawbacks of the bottom-up approach.
Both approaches provide more than enough future challenges and are equally scientifically interesting. However, in terms of future device application potential, the top-down approach has several advantages over the bottom-up approach.
Continue reading: Manipulating Nanowires with Atomic Force Microscopy
References and Further Reading
Dasgupta, N. P., et al. (2014). 25th anniversary article: semiconductor nanowires–synthesis, characterization, and applications. Advanced materials, 26(14), 2137-2184. https://onlinelibrary.wiley.com/doi/abs/10.1002/adma.201305929
Peng, K. Q., et al. (2006). Fabrication of single‐crystalline silicon nanowires by scratching a silicon surface with catalytic metal particles. Advanced Functional Materials, 16(3), 387-394. https://onlinelibrary.wiley.com/doi/10.1002/adfm.200500392
Chen, J. T., et al. (2008). CuO nanowires synthesized by thermal oxidation route. Journal of Alloys and Compounds, 454(1-2), 268-273. http://dx.doi.org/10.1016/j.jallcom.2006.12.032
Park, H., et al. (2013). Graphene cathode based ZnO nanowire hybrid solar cells. Nano letters, 13(1), 233-239. https://doi.org/10.1021/nl303920b
Lv, Z., et al. (2018). Editable supercapacitors with customizable stretchability based on mechanically strengthened ultralong MnO2 nanowire composite. Advanced Materials, 30(2), 1704531. https://onlinelibrary.wiley.com/doi/abs/10.1002/adma.201704531
Wolfsteller, A., et al. (2010). Comparison of the top-down and bottom-up approach to fabricate nanowire-based silicon/germanium heterostructures. Thin Solid Films, 518(9), 2555-2561. http://dx.doi.org/10.1016/j.tsf.2009.08.021
Disclaimer: The views expressed here are those of the author expressed in their private capacity and do not necessarily represent the views of AZoM.com Limited T/A AZoNetwork the owner and operator of this website. This disclaimer forms part of the Terms and conditions of use of this website.