Nanotechnology has emerged as a ground-breaking field with vast potential in various industries, ranging from medicine and electronics to environmental science and energy. At the heart of this revolutionary technology lies the nanoparticle, with dimensions on the nanoscale.
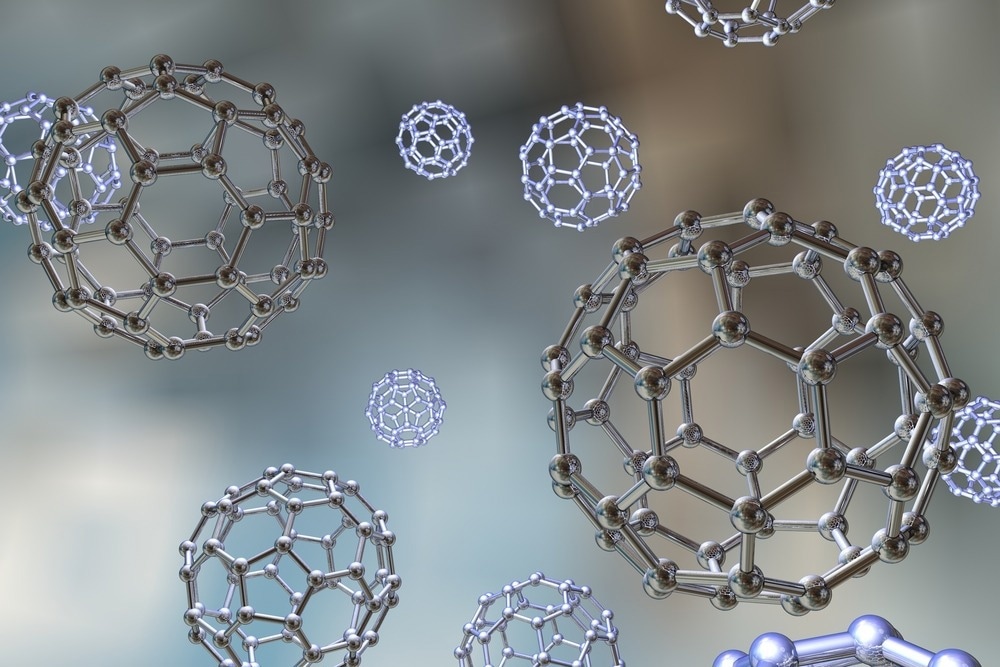
Image Credit: Kateryna Kon/Shutterstock.com
Nanoparticles exhibit unique properties that differ from their bulk counterparts, making them highly desirable for numerous applications. However, understanding and analyzing nanoparticles present unique challenges due to their small size and complex nature.
Characterizing Nanoparticles: Why Does Analysis Matters?
The properties of nanoparticles, such as size, shape, composition, and surface characteristics, significantly influence their behavior and functionality. Accurate characterization is crucial for optimizing nanoparticle synthesis, designing tailored applications, and ensuring safety in various fields.
Physical characterization techniques, such as Dynamic Light Scattering (DLS), Electron Microscopy (TEM/HR-TEM), Atomic Force Microscopy (AFM), BET theory, and X-ray diffraction (XRD), provide insights into nanoparticle size, morphology, and crystalline structure. Chemical characterization methods, such as Nuclear Magnetic Resonance spectroscopy (NMR), Inductively Coupled Plasma Mass Spectroscopy (ICP-MS), and Fourier Transform Infrared Spectroscopy (FTIR) help determine the elemental composition, molecular structures, and interactions of nanoparticles.
Electron Microscopy (TEM/HR-TEM)
Transmission electron microscopy (TEM) is a high-resolution microscopy technique that uses a focused electron beam for imaging. The contrast obtained in TEM imaging depends on the sample thickness and material, with heavier atoms scattering more electrons and producing more contrast. TEM is capable of providing dimensionally accurate images of the nanoparticles with a 3% margin of error.
Atomic Force Microscopy (AFM)
It is an advanced technology capable of generating detailed 3D images of surfaces with a resolution up to a fraction of an angstrom. It uses a sharp fragile tip connected to a cantilever to scan through the particle. AFM measures the deflection in the cantilever due to attractive or repulsive forces between the tip and the surface, quantified using a laser beam.
Counting on the advantages, it does not require surface modifications or coatings before imaging, making it suitable for analyzing small nanoparticles (up to 6 nm) without specialized treatment. Low-density materials with poor contrast in electron microscopy can particularly be characterized using the technique. AFM provides resolution comparable to transmission electron microscopy (TEM) but at a lower cost. These features make AFM a valuable tool for nanoparticle characterization and offer insights into their surface properties.
BET (Brunauer-Emmett-Teller)
The BET theory, coined by Stephen Brunauer, Paul Hugh Emmett, and Edward Teller, is a model used in physical chemistry to explain how gas molecules adhere to solid surfaces. At its core, the BET theory assumes that gas molecules can adhere to the surface of solid material in multiple layers. The theory is expressed through the BET equation, which describes the relationship between gas pressure, adsorbed gas quantity, and surface properties of the adsorbent. The BET theory is valuable in characterizing the surface area and porosity of materials, and it has been used in many experimental techniques, including nitrogen adsorption isotherms.
X-Ray Diffraction
XRD is utilized extensively for analyzing nanoparticles, providing crucial insights into their crystalline structure, phase characteristics, lattice parameters, and crystallite size. The estimation of crystallite size is often carried out using the Scherrer method, which involves measuring the peak broadening in the spectrum. This technique offers the advantage of generating statistically representative and volume-averaged values when applied to dried powdered samples after colloidal solutions have been prepared.
However, for particles smaller than 3 nm, the broadening increases extensively to a limit that it is not a useful technique anymore. In general, the crystallite size obtained from XRD tends to be larger than the magnetic size. This is due to the presence of smaller magnetic domains with aligned magnetic moments within a particle, even if the particle has one large domain. Contrarily, when examining samples with large particle sizes and smaller crystallite sizes, the results of TEM differ from that of an XRD and exhibit large and accurate nanoparticle sizes.
ICP-MS (Inductively Coupled Plasma Mass Spectroscopy)
It is an analytical technique used to identify and quantify the elemental composition of samples, including metals and certain non-metals within a specific atomic mass range. Its sensitivity is a key advantage, allowing for detecting elements in trace amounts. In fact, ICP-MS can achieve detection limits as sophisticated as parts per trillion to parts per quadrillion for many elements.
The process of ICP-MS involves nebulizing liquid samples and exposing them to a high-temperature argon plasma, resulting in the ionization of the elements through electron excitation. Subsequently, ionized atoms are separated from neutral particles and detected by a mass spectrometer. Different elements and their concentrations can be determined by measuring the mass-to-charge ratio of the ions.
Fourier Transform Infrared Spectroscopy (FTIR)
FTIR is a method that measures the absorption of electromagnetic radiation within the infrared range of 4000-400 cm-1. Upon absorbing the IR radiation, the molecule undergoes changes in its dipole moment and becomes IR active. By analyzing the recorded spectrum, valuable information about bond strength, nature, specific functional groups, molecular structures, and interactions can be obtained. FTIR spectroscopy aids in understanding these aspects of nanoparticles.
Nuclear Magnetic Resonance (NMR) Spectroscopy
A technique used to determine and quantify the molecular structure of nanoscale materials. It also helps in determining the phases and their diffusion potential. It counts on the resonance phenomenon demonstrated by the nuclei when subjected to a strong magnetic field, generating an energy difference between the two opposite spin states. This difference in energy is detected and analyzed to gain insight into the chemistry of the nanoparticles.
Despite significant advancements in nanoparticle analysis techniques, several challenges persist. The characterization of complex nanoparticle mixtures, identifying trace amounts of nanoparticles, and understanding their behaviour in dynamic environments are ongoing areas of research. The future of nanoparticle analysis holds promise. Advancements in machine learning, artificial intelligence, and big data analysis can assist in rapidly processing vast amounts of data obtained from nanoparticle analysis, facilitating the extraction of valuable insights.
References and Further Reading
Mourdikoudis, Stefanos, et al. (2018). Characterization techniques for nanoparticles: comparison and complementarity upon studying nanoparticle properties. Nanoscale. pp. 12871-12934. https://pubs.rsc.org/en/content/articlelanding/2018/nr/c8nr02278j
Upadhyay, Sneha, et al. (2016). Influence of crystallite size on the magnetic properties of Fe3O4 nanoparticles. Journal of Alloys and Compounds. pp. 478-485. https://www.sciencedirect.com/science/article/abs/pii/S0925838816309343
L. Reimer et al. (2009). Transmission Electron Microscopy Physics of Image Formation, Springer, New York, pp. 1–15. https://link.springer.com/book/10.1007/978-0-387-40093-8
Walton, Krista et al (2007). Applicability of the BET method for determining surface areas of microporous metal−organic frameworks. Journal of the American Chemical Society. pp. 8552-8556. https://pubs.acs.org/doi/10.1021/ja071174k
Disclaimer: The views expressed here are those of the author expressed in their private capacity and do not necessarily represent the views of AZoM.com Limited T/A AZoNetwork the owner and operator of this website. This disclaimer forms part of the Terms and conditions of use of this website.