Antimicrobial resistance and increasing healthcare costs have inspired researchers to create innovative and effective antimicrobial therapies. Much focus has been placed upon 'nanoparticle-based antimicrobials', as nanoparticles offer distinct benefits compared to traditional chemical antimicrobial agents with multidrug resistance.
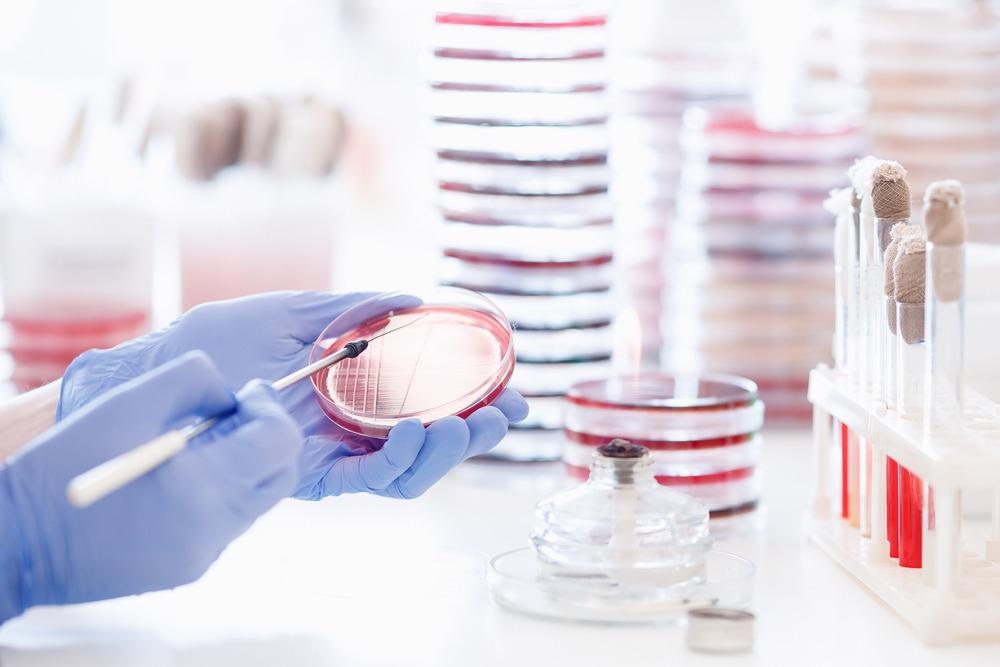
Image Credit: Parilov/Shutterstock.com
Silver nanoparticles (AgNPs) include amphiphilic hyperbranched macromolecules, making them an effective antimicrobial surface coating agent. These nanoparticles can even be modified to increase efficiency, enabling their use in various fields, particularly healthcare.
Nanoparticles synthesized through green nanotechnology, however, are an area attracting growing interest.
Green nanotechnology aims to eliminate or reduce the pollution caused by conventional methods used for their synthesis. Furthermore, environmental impacts in the product chain are estimated and mitigated, resulting in this approach becoming highly favorable for a wide range of biomedical and biotechnological applications.
The production of AgNPs through green nanotechnology is a simple, cost-effective, high-yield synthesis and an eco-friendly procedure. It was introduced to the synthesis of nanoparticles without using toxic chemicals and avoids the production of undesirable toxic products.
A recent study illustrates the production of AgNPs through green technology and its coating by a quaternary ammonium salt, benzalkonium chloride (BAC), with synthesized AgNPs characterized by TEM and Dynamic Light Scattering (DLS).
Antimicrobial screening of AgNPs and BAC-coated AgNPs was performed against a range of gram-positive (gm+) bacteria and gram-negative (gm−) bacteria by colony-forming units, β-glucosidase activity, and a zone of inhibition.
Methodology
AgNPs were synthesized with slight modifications. Firstly, fresh neem leaves were washed using double-distilled water and then air-dried at room temperature. Secondly, 50 gm neem leaves were soaked in 250 mL double-distilled water and boiled for 1 hour. The obtained extract was cooled down and filtered using Whatman filter paper No. 1.
Then, Azadirachta indica leaf extract and 1 mM AgNO3 were mixed in a 1:10 ratio. The solution was placed on a magnetic stirrer in an ice bath for 30 minutes at room temperature in a dark chamber to inhibit the photo-activation of silver nitrate.
Ag+ reduced to Ag0 with the solution turning brown, implying the formation of AgNPs. Benzalkonium chloride (0.1% w/v) was coated on the surface of the resulting AgNPs in an Erlenmeyer glass at room temperature in a dark chamber on a magnetic stirrer.
The morphology, dimensions, and size of AgNPs were obtained. TEM analysis samples were prepared through drop-coating of diluted NP solution on carbon-coated copper grids at standard atmospheric conditions before characterizing AgNPs.
The bactericidal effect of AgNPs against four different clinical pathogenic bacteria—Escherichia coli, Pseudomonas aeruginosa, Bacillus subtilis, and Streptococcus pneumoniae—was analyzed.
Through ultrasonication, AgNPs were dispersed in pre-sterilized millipore water. Desired concentrations (0.0625, 0.1250, 0.250, 0.500, and 1.0 mM) for bacterial effects analysis were formulated with the aqueous dispersions of AgNPs
Results
Figure 1 displays the TEM image of AgNPs, representing the fineness of the particles with a mean diameter of 30 nm. Dynamic light scattering was employed to identify the size distribution profile of AgNPs. From Figure 2, it can be seen that the average mean size of AgNPs was 30 nm.
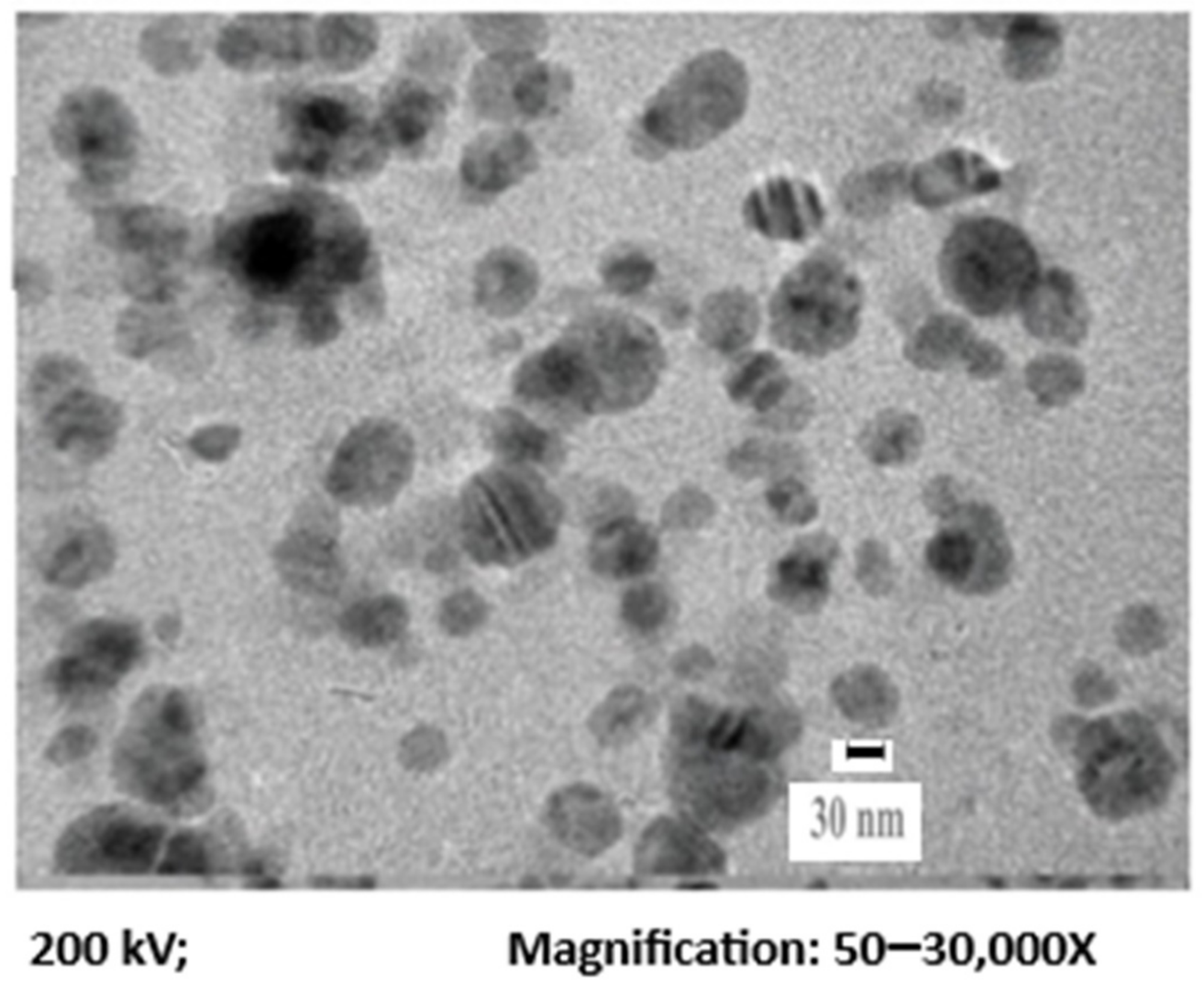
Figure 1. TEM of the synthesized AgNPs. Image Credit: Ansari & Alshanberi, 2021
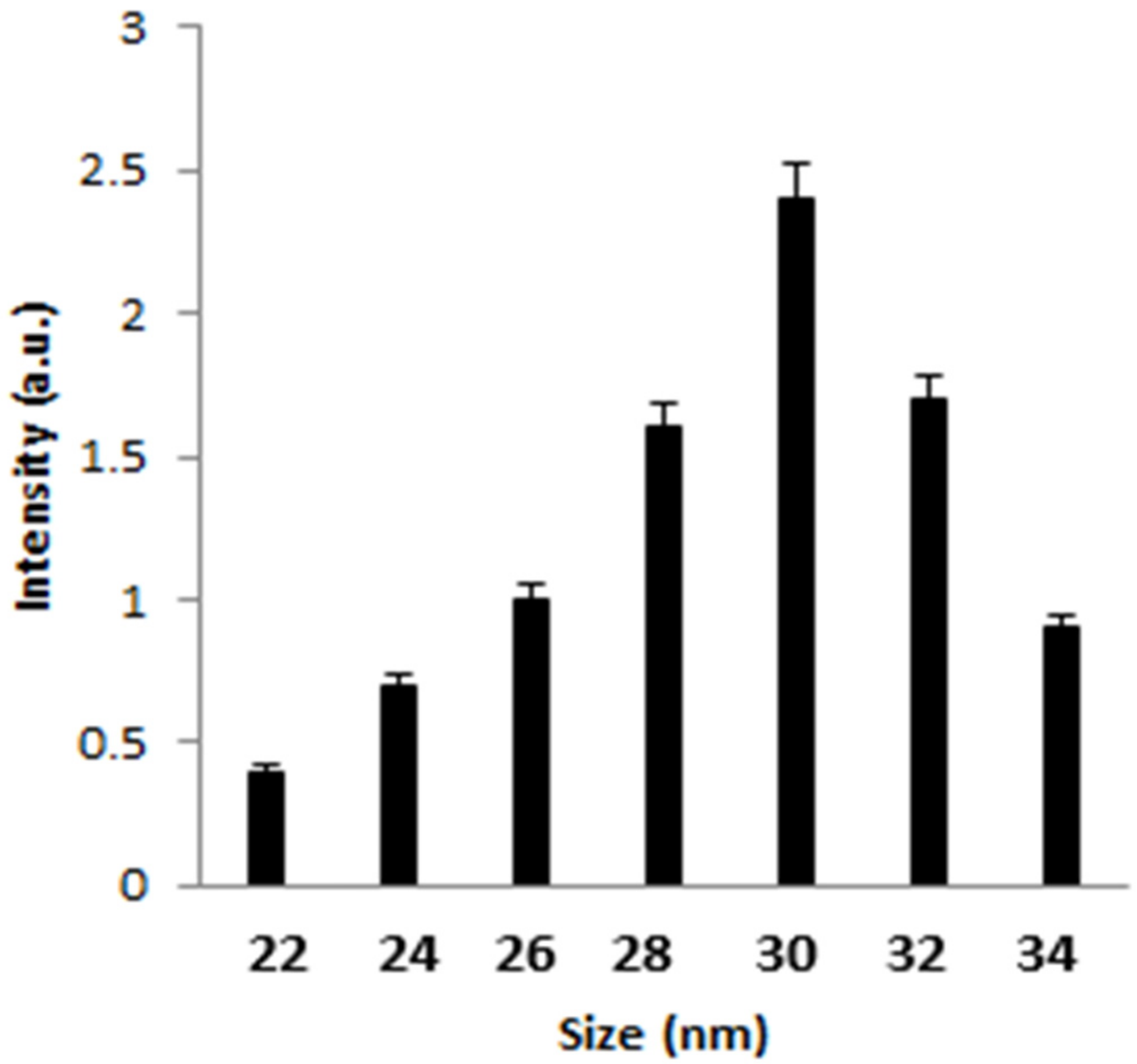
Figure 2. DLS of the synthesized AgNPs. Image Credit: Ansari & Alshanberi, 2021
Antibacterial assays found that increased concentration of AgNPs led to a considerable reduction in CFU numbers. For instance, at 0.25 mM AgNPs, 22 × 109 CFU were formed. However, for benzalkonium chloride-coated AgNPs, this figure was reduced to 14 × 109 CFU under similar experimental conditions (see Figure 3).
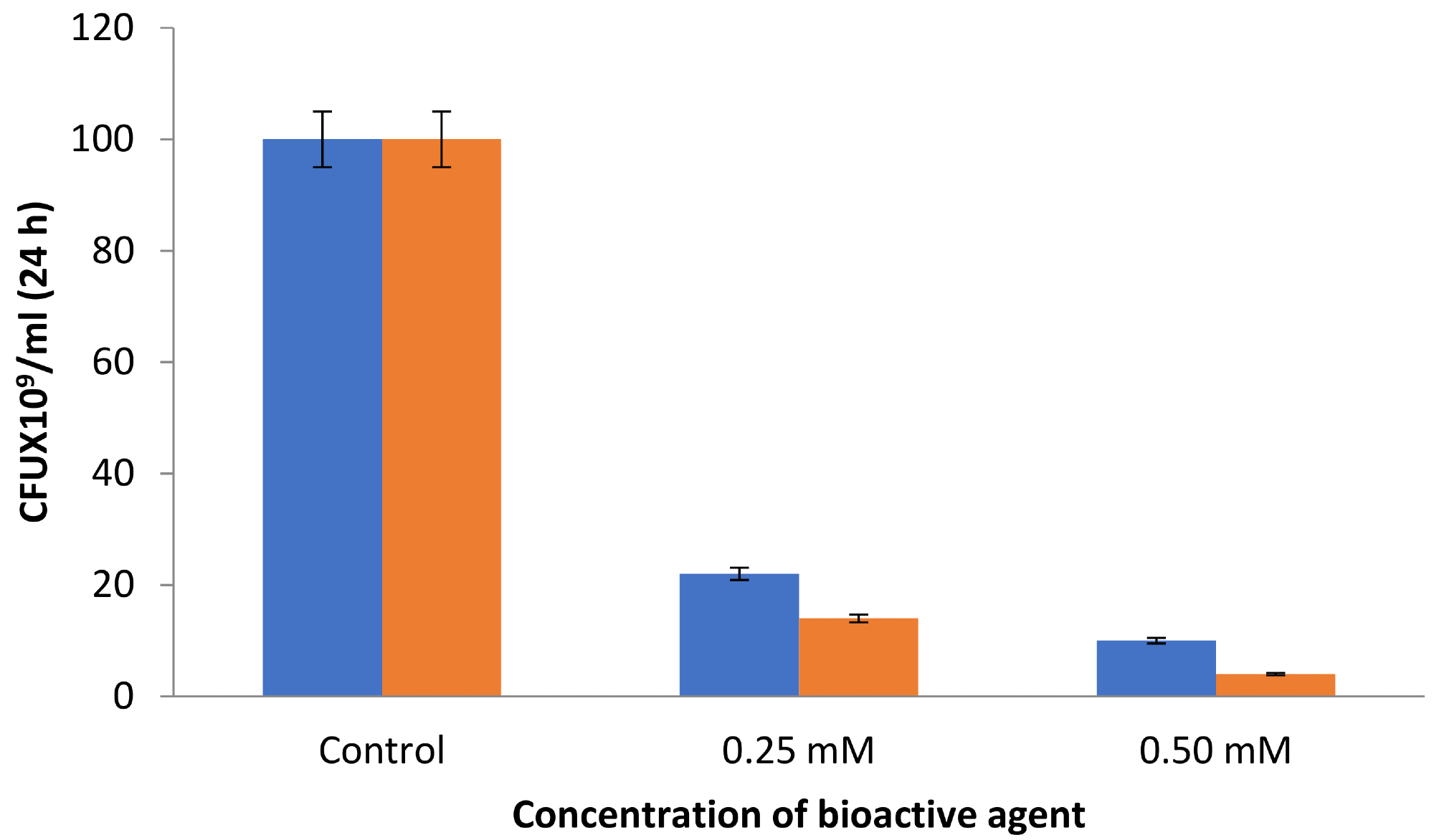
Figure 3. CFU by the synthesized AgNPs (blue) and BAC-coated AgNPs (orange). Image Credit: Ansari & Alshanberi, 2021
β-glucosidase activity was observed under different AgNPs concentrations. Researchers found a substantial increase in the enzymatic activity of β-glucosidase beyond 0.0625 mM and up to 0.5 mM concentration of AgNPs and BAC–AgNPs (see Figure 4)
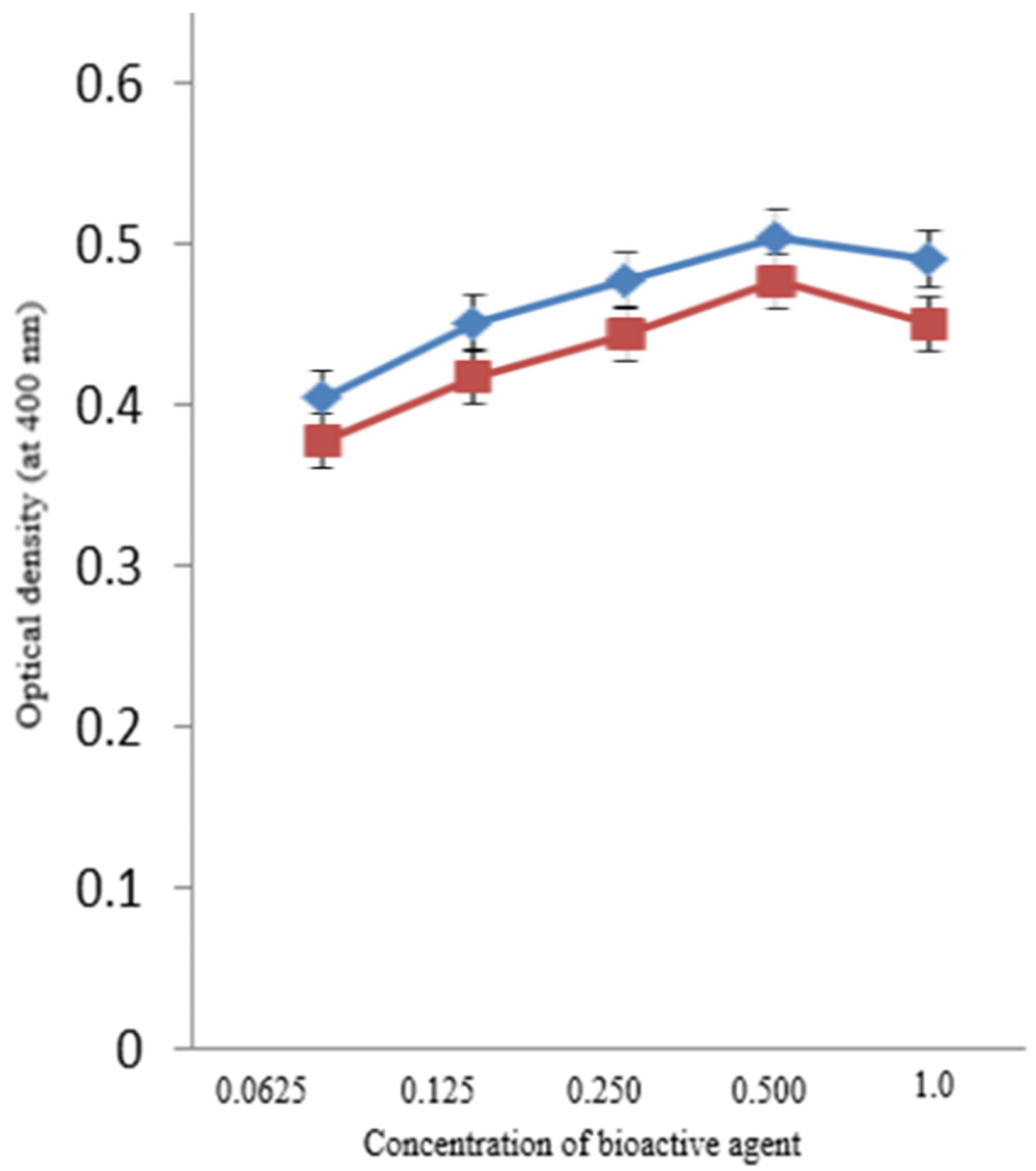
Figure 4. Activity of β-glucosidase with the synthesized AgNPs (blue) and BAC-coated AgNPs (red). Image Credit: Ansari & Alshanberi, 2021
No additional changes in activity were observed beyond this concentration.
Table 1 indicates the zone of inhibition of AgNPs and BAC–AgNPs against E. coli, P. aeruginosa, B. subtilis, and S. pneumoniae at 0.25 and 0.50 mM concentration of these bioactive agents, respectively.
Table 1. Zone of inhibition (diameter, cm) of antibacterial test of AgNPs and Benzalkonium chloride (BAC)-coated AgNPs. Source: Ansari & Alshanberi, 2021
Concentration (mM) |
E. coli |
B. subtilis |
P. aeruginosa |
S. pneumonia |
AgNPs |
BAC–AgNPs |
AgNPs |
BAC–AgNPs |
AgNPs |
BAC–AgNPs |
AgNPs |
BAC–AgNPs |
0.25 |
3.45 ± 0.85 |
3.56 ± 0.54 |
4.28 ± 0.39 |
4.40 ± 0.58 |
3.36 ± 1.2 |
3.47 ± 1.34 |
3.44 ± 0.96 |
3.62 ± 0.68 |
0.50 |
4.28 ± 0.73 |
4.40 ± 0.68 |
4.36 ± 0.98 |
4.44 ± 0.77 |
4.28 ± 0.98 |
4.37 ± 1.55 |
3.78 ± 0.27 |
3.91 ± 0.37 |
An increase in the concentration of these bioactive agents to 0.5 mM led to a significant decrease in the ZOI for BAC–AgNPs than AgNPs. E. coli ZOI was found to be 4.28 and 4.40 cm, while S. pneumoniae ZOI was 3.78 and 3.91 cm for AgNPs and BAC–AgNPs, respectively under similar incubation conditions.
Conclusion
In this study, the synthesis of AgNPs using neem leaf meets all the conditions of a 100% green chemical process. This includes an environmentally friendly, fast, and green approach that is economical and does not use reducing agents or external stabilizers.
Researchers found that the use of benzalkonium chloride as a quaternary ammonium compound led to major improvements in the antibacterial activity of the silver nanoparticles produced from the neem leaves.
Superior antimicrobial efficacy was achieved against bacterial strains like E. coli, P. aeruginosa, B. subtilis, and S. pneumoniae. The persistent antibacterial activity of such nanoparticles against other microorganisms may be studied to extend their use to biomedical, environmental, and biotechnological sectors. This study will also have major implications for the development of other nanoparticles by clean and green technologies.
Continue reading: An Overview of the Synthesis and Application of Green Nanoparticles.
Journal Reference:
Ansari, S A & Alshanberi, A M (2021) Clinical Application of Silver Nanoparticles Coated by Benzalkonium Chloride. Coatings, 11(11), p. 1382. Available at: https://doi.org/10.3390/coatings11111382.
References and Further Reading
- Nikaido, H (2009) Multidrug resistance in bacteria. Annual Review of Biochemistry, 78, pp. 119–146. doi.org/10.1146/annurev.biochem.78.082907.145923.
- Talebian, S., et al. (2020) Nanotechnology-based disinfectants and sensors for SARS-CoV-2. Nature Nanotechnology, 15, pp. 618–621. doi.org/10.1038/s41565-020-0751-0.
- Wang, L., et al. (2017) The antimicrobial activity of nanoparticles: Present situation and prospects for the future. International Journal of Nanomedicine, 12, pp. 1227–1249. doi.org/10.2147/IJN.S121956.
- Varier, K. M., et al. (2019) Nanoparticles: Antimicrobial applications and its prospects. In: Advanced Nanostructured Materials for Environmental Remediation; Environmental Chemistry for a Sustainable World; Naushad, M., et al., Eds.; Springer: Cham, Switzerland; Volume 25, pp. 321–355.
- Li, D., et al. (2020) Antibacterial therapeutic agents composed of functional biological molecules. Journal of Chemistry, 2020, p. 6578579. doi.org/10.1155/2020/6578579.
- Hooper, D C (2001) Mechanisms of action of antimicrobials: Focus on fluoroquinolones. Clinical Infectious Diseases, 32, pp. S9–S15. doi.org/10.1086/319370.
- Bazaka, K., et al. (2015) Antibacterial surfaces: Natural agents, mechanisms of action, and plasma surface modification. RSC Advances, 5, pp. 48739–48759. doi.org/10.1039/C4RA17244B.
- Herman, A & Herman, A P (2014) Nanoparticles as antimicrobial agents: Their toxicity and mechanisms of action. Journal of Nanoscience and Nanotechnology, 14, pp. 946–957. doi.org/10.1166/jnn.2014.9054.
- Almatar, M., et al. (2018) The role of nanoparticles in the inhibition of multidrug-resistant bacteria and biofilms. Current Drug Delivery, 15, pp. 470–484. doi.org/10.2174/1567201815666171207163504.
- Gudikandula, K & Maringanti, S C (2016) Synthesis of silver nanoparticles by chemical and biological methods and their antimicrobial properties. Journal of Experimental Nanoscience, 11, pp. 714–721. doi.org/10.1080/17458080.2016.1139196.
- Franci, G., et al. (2015) Silver Nanoparticles as Potential Antibacterial Agents. Molecules, 20, pp. 8856–8874. doi.org/10.3390/molecules20058856.
- Siddiqi, K. S., et al. (2018) A review on biosynthesis of silver nanoparticles and their biocidal properties. Journal of Nanobiotechnology, 16, pp. 14–22. doi.org/10.1186/s12951-018-0334-5.
- Yaqoob, A. A., et al. (2020) Silver nanoparticles: Various methods of synthesis, size affecting factors and their potential applications–a review. Applied Nanoscience, 10, pp. 1369–1378. doi.org/10.1007/s13204-020-01318-w.
- Islam, A., et al. (2021) A critical review on silver nanoparticles: From synthesis and applications to its mitigation through low-cost adsorption by biochar. Journal of Environmental Management, 281, pp. 111918–111932. doi.org/10.1016/j.jenvman.2020.111918.
- Syafiuddin, A., et al. (2017) A review of silver nanoparticles: Research trends, global consumption, synthesis, properties, and future challenges. Journal of the Chinese Chemical Society, 64, pp. 732–756. doi.org/10.1002/jccs.201700067.
- Verma, A., et al. (2019) Green Nanotechnology: Advancement in phytoformulation research. Medicines, 6, pp. 39–49. doi.org/10.3390/medicines6010039.
- Patra, J K & Baek, K-H (2014) Green nanobiotechnology: Factors affecting synthesis and characterization techniques. Journal of Nanomaterials, 2014, p. 417305. doi.org/10.1155/2014/417305.
- Ansari, S. A., et al. (2012) Cost effective surface functionalization of silver nanoparticles for high yield immobilization of Aspergillus oryzae β-galactosidase and its application in lactose hydrolysis. Process Biochemistry, 47, pp. 2427–2433. doi.org/10.1016/j.procbio.2012.10.002.
- Roy, A., et al. (2019) Green synthesis of silver nanoparticles: Biomolecule-nanoparticle organizations targeting antimicrobial activity. RSC Advances, 9, pp. 2673–2702. doi.org/10.1039/C8RA08982E.
- Ahmad, S., et al. (2019) Green nanotechnology: A review on green synthesis of silver nanoparticles—An ecofriendly approach. International Journal of Nanomedicine, 14, pp. 5087–5107. doi.org/10.2147/IJN.S200254.
- Javed, R., et al. (2020) Role of capping agents in the application of nanoparticles in biomedicine and environmental remediation: Recent trends and future prospects. Journal of Nanobiotechnology, 18, pp. 172–185. doi.org/10.1186/s12951-020-00704-4.
- Verma, A & Mehata, M S (2016) Controllable synthesis of silver nanoparticles using Neem leaves and their antimicrobial activity. Journal of Radiation Research and Applied Sciences, 9, pp. 109–115. doi.org/10.1016/j.jrras.2015.11.001.
- Banerjee, P., et al. (2014) Leaf extract mediated green synthesis of silver nanoparticles from widely available Indian plants: Synthesis, characterization, antimicrobial property and toxicity analysis. Bioresources and Bioprocessing, 1, pp. 3–10. doi.org/10.1186/s40643-014-0003-y.
- Saware, K & Venkataraman, A (2014) Biosynthesis and Characterization of Stable Silver Nanoparticles Using Ficus religiosa Leaf Extract: A Mechanism Perspective. Journal of Cluster Science, 25, pp. 1157–1171. doi.org/10.1007/s10876-014-0697-1.
- Goudarzi, M., et al. (2016) Biosynthesis and characterization of silver nanoparticles prepared from two novel natural precursors by facile thermal decomposition methods. Scientific Reports, 6, pp. 32539–32549. doi.org/10.1038/srep32539.
- Jadoun, S., et al. (2021) Green synthesis of nanoparticles using plant extracts: A review. Environmental Chemistry Letters, 19, pp. 355–374. doi.org/10.1007/s10311-020-01074-x.
- Kim, M., et al. (2018) Widely used benzalkonium chloride disinfectants can promote antibiotic resistance. Applied and Environmental Microbiology, 84, pp. 17–25. doi.org/10.1128/AEM.01201-18.
- Ahmed, S., et al. (2016) Green synthesis of silver nanoparticles using Azadirachta indica aqueous leaf extract. Journal of Radiation Research and Applied Sciences, 9, pp. 1–7. doi.org/10.1016/j.jrras.2015.06.006.
- Ansari, S. A., et al. (2017) Antibacterial activity of iron oxide nanoparticles synthesized by co-precipitation technology against Bacillus cereus and Klebsiella pneumoniae. Polish Journal of Chemical Technology, 19, pp. 110–115. doi.org/10.1515/pjct-2017-0076.
- Khajav, R., et al. (2007) The antimicrobial effect of benzalkonium chloride on some pathogenic microbes observed on fibers of acrylic carpet. Pakistan Journal of Biological Sciences, 10, pp. 598–601. doi.org/10.3923/pjbs.2007.598.601.
- Mazur, P., et al. (2020) Synergistic ROS-associated antimicrobial activity of silver nanoparticles and gentamicin against Staphylococcus epidermidis. International Journal of Nanomedicine, 15, pp. 3551–3562. doi.org/10.2147/IJN.S246484.
- Krce, L., et al. (2020) Probing the mode of antibacterial action of silver nanoparticles synthesized by laser ablation in water: What fluorescence and AFM data tell us. Nanomaterials, 10, pp. 61–72. doi.org/10.3390/nano10061040.
- Koschevic, M. T., et al. (2021) Antimicrobial activity of bleached cattail fibers (Typha domingensis) impregnated with silver nanoparticles and benzalkonium chloride. Journal of Applied Polymer Science, 138, pp. 55–67. doi.org/10.1002/app.50885.