Fossil fuel supplies are diminishing, and burning them for energy has brought us to the brink of a climate crisis. While renewable energy from the sun, wind, tides, and ground has risen to meet the challenge of replacing fossil fuels, there is still another potential energy source that has not yet been fully tapped. So-called ‘blue energy’ can be harvested from the chemical reactions between water of different salinities, and nanoporous membranes are the key to unlocking it.
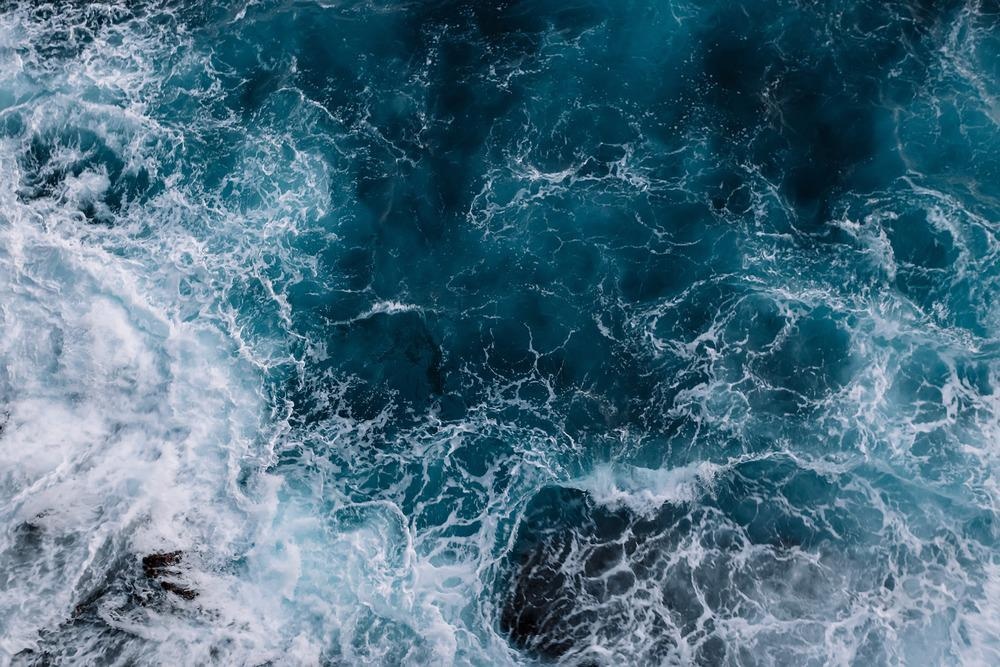
Image Credit: Ivan Kurmyshov/Shutterstock.com
Fossil fuel supplies are diminishing, and burning them for energy has brought us to the brink of a climate crisis. While renewable energy from the sun, wind, tides, and ground has risen to meet the challenge of replacing fossil fuels, there is still another potential energy source that has not yet been fully tapped. So-called 'blue energy' can be harvested from the chemical reactions between water of different salinities, and nanoporous membranes are the key to unlocking it.
In a recent paper published in the Royal Society of Chemistry's journal, Chemical Science, an international team of researchers outlined the role of nanoporous membranes in blue energy production. The authors are from the Universidad Nacional de La Plata in Argentina, and the Technische Universität Darmstadt and GSI Helmholtz Centre for Heavy Ion Research in Germany.
The Helmholtz Centre for Heavy Ion Research is a one-of-its-kind particle accelerator in Darmstadt, Germany, operated by the country's Society for Heavy Ion Research (GSI). In it, researchers from around the world conduct experiments with heavy ions.
How Does Mixing Water Create Energy?
Blue energy is acquired by exploiting the osmotic pressure difference between two sources of water that have different salinities (salt levels). Osmotic pressure is a measure of how much a solution can take in a pure solvent by osmosis. Water sources with different osmotic pressures permeate membranes at different rates.
This difference is a harvestable source of energy referred to as salinity gradient energy (SGE) or blue energy. Blue energy comes from the capture and conversion of Gibbs free energy (a thermodynamic potential).
This unexpected source was first suggested in 1954 where scientists compared the energy potential of a river mixing with the sea with a 680 ft (207 m) waterfall.
When freshwater mixes with salty water at the confluence of rivers and sea, Gibbs free energy is released in entropic changes that occur naturally at the chemical level. Today, scientists estimate that around 0.8 kW h/m–3 of water is harvestable from naturally occurring blue energy sources like these.
The first experimental technique developed for harnessing blue energy is reverse electrodialysis (RED). RED systems employed stacked cation and anion exchange membranes to capture blue energy from alternating seawater and river water.
In 1974, researchers developed a new technique that used osmotic membranes: pressure retarded osmosis (PRO).
In both systems, the main limitation to fully realizing blue energy's potential was the availability of suitable membranes that could be reliably synthesized at scale.
The potential use of ion-selective dense membranes to create and harvest energy from different osmotic pressures is already well established. However, blue energy systems have yet to be scaled to industrial levels due to technological limitations in synthesizing suitable membranes.
Blue Energy- Salinity gradient power | ACCIONA Imnovation
Video Credit: ACCIONA/YouTube.com
Nanoporous Membranes to Unlock Blue Energy's Potential
The development of blue energy has been inextricably linked with the development of more efficient membranes that can be synthesized at scale. Now, a new generation of nanoporous membranes (materials whose pores measure between 0.1 and 100 nm) has entered the blue energy domain.
These materials can work as blue energy harvesters because charged groups form on the surface of their nanoscale pores. However, early nanoporous membranes proposed for blue energy harvesting were limited in terms of their flux ion efficiency.
Blue energy research is therefore focused on developing nanoporous membranes that allow a large number of ions (charged atoms or molecules) to flow through them.
Nanoporous membranes with high ion flux and the appropriate selectivity to harness blue energy are the prize that researchers worldwide are striving toward.
Materials have been proposed that meet these requirements, but synthesizing nanoporous membranes is still a new field. Therefore, large-scale production is as yet still only a theoretical possibility, and work needs to be done to make it a reality.
New Materials and New Techniques to Take Blue Energy Forward
In a new paper, scientists show that utilizing nanoporous membranes with tailored ion transport properties could unlock high-efficiency blue energy harvesting systems that work at scale.
The authors are motivated by a "learning from nature" approach. They celebrate biomimetic techniques for blue energy capture based on solid-state nanochannels (SSNs). The optimum technique, they argue, is nanofluidic reverse electrodialysis, or NRED, that makes use of the new generation of nanoporous membranes.
NRED enables controlled mixing in nanoporous membranes instead of the uncontrolled mixing in dense membranes used in traditional RED. Unlike dense membranes, nanoporous membranes combine low resistance to ions (high ion flux) with good ion selectivity.
This technique establishes the concentration gradient from electrostatic interactions attracting counterions and expelling co-ions when an SSN-containing nanoporous membrane is exposed to an electrolyte solution.
Scaling Up Synthesis of Nanoporous Membranes
The key to unlocking the potential of nanoporous membranes in blue energy production is developing effective synthesizing techniques for them. No method has emerged as the most effective so far, but some success has been possible with ion beams, electron beams, and electrochemical techniques.
One key challenge for producing scalable NRED devices is the difficulty of making the leap from single-pore to multi-channel operation.
The authors of the latest study summarizing the field identified three main barriers to scaling up NRED technology to industrial levels:
- Making systems work over realistic working areas (bigger scale and higher capacity than laboratory conditions)
- Creating full, multi-channel NRED cells
- Improving the mechanical and antifouling properties of nanoporous membranes
The paper argues that an interdisciplinary effort will be required to overcome these challenges. Engineers, materials scientists, nanotechnologists, physical chemists, and electrical chemists all have an important role to play in the future of blue energy.
Continue reading: IPCC Editorial Series: Industrial Response to Climate Change.
References and Further Reading
Laucirica, G. et al. (2021). Nanofluidic osmotic power generators – advanced nanoporous membranes and nanochannels for blue energy harvesting. Chemical Science. Available at: https://doi.org/10.1039/D1SC03581A
Norman, R. S. (1974). Water Salination: A Source of Energy. Science. Available at: https://doi.org/10.1126/science.186.4161.350
Pattle, R. E. (1954). Production of Electric Power by mixing Fresh and Salt Water in the Hydroelectric Pile. Nature. Available at: https://doi.org/10.1038/174660a0
Tedesco, M. et al. (2015). Reverse electrodialysis with saline waters and concentrated brines: A laboratory investigation towards technology scale-up. Journal of Membrane Science. Available at: https://doi.org/10.1016/j.memsci.2015.05.020
Tian, H., Y. Wang, Y. Pei, and J. C. Crittenden (2020). Unique applications and improvements of reverse electrodialysis: A review and outlook. Applied Energy. Available at: https://doi.org/10.1016/j.apenergy.2019.114482
Zhang, L., and X. Cheng (2013). Nanofluidics for Giant Power Harvesting. Angewandte Chemie. Available at: https://doi.org/10.1002/anie.201302707
Disclaimer: The views expressed here are those of the author expressed in their private capacity and do not necessarily represent the views of AZoM.com Limited T/A AZoNetwork the owner and operator of this website. This disclaimer forms part of the Terms and conditions of use of this website.