AZoNano speaks with Dr. Georgios 'Yorgos' Katsikis about his involvement in new research that employs nanofluidics to assess the DNA content of viral vectors, a critical component of gene therapy. Integrating this approach into a biomanufacturing workflow could help enhance clinical safety and therapeutic efficiency via quality control.
Please could you introduce yourself, and how you became involved in this field of research?
I am a Postdoctoral Associate at Massachusetts Institute of Technology (MIT). I started my career as a mechanical engineer researching fluid dynamics phenomena in aircraft engines. Yet, during my Ph.D. at Stanford University, I discovered I could use my background in fluid dynamics down to the micro- and nanoscale to study the world of biology.
In my postdoc, I led a collaboration between MIT, Biomarin Pharmaceutical and CEA-Leti to develop a nanofluidic platform for high-throughput measurements of viruses for gene therapy.
Could you give an overview of your most recent publication?
In our work, published in ACS Nano Letters we developed a nanofluidic weight scale to quantify the DNA content of Adeno-Associated Virus (AAV) vectors.
AAV is an immensely popular vector for gene therapy due to its non-pathogenicity, and versatility to treat various diseases. Therapeutic DNA is packed inside AAV in biomanufacturing; however, quantifying this DNA content is laborious and time-consuming when using traditional biochemical methods. Our platform is based on nanomechanical resonators and has the potential to provide real-time quality control during biomanufacturing.
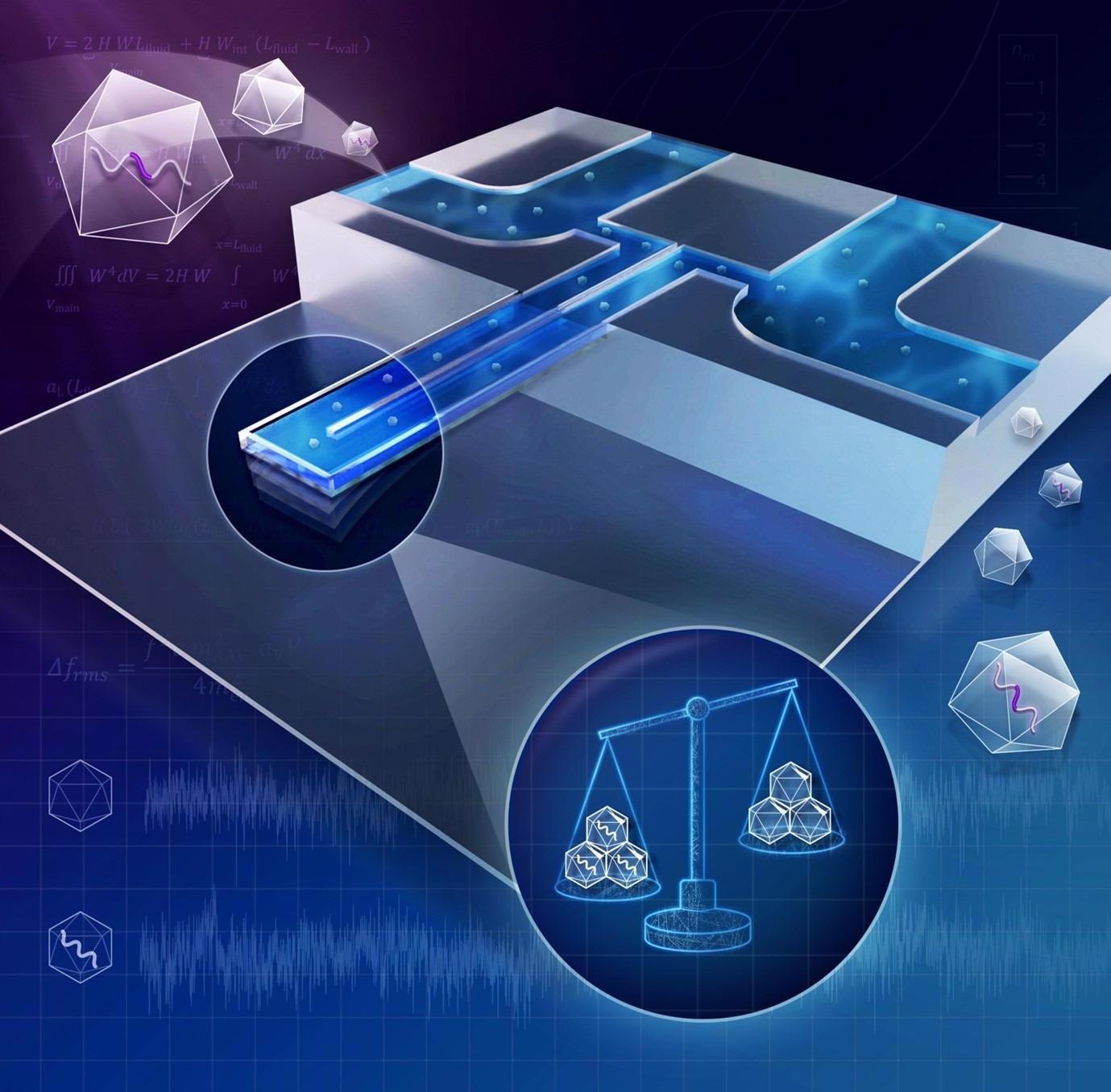
© Betsy Skrip/ MIT
Could you define ‘Nanomechanical Resonators’ and their role in this project?
When plucking the thinnest guitar string, a sound with the least bass is produced, resulting from the string vibrating at its resonant frequency of around 300 Hz. In that sense, the guitar strings are ‘macromechanical resonators’; if you pluck the thickest string, you will get a mostly bass sound of a frequency of around 80 Hz. The harder, thicker or heavier a string is, the lower its resonant frequency becomes.
Our nanomechanical resonator is a water-filled miniature tube that, similar to a guitar string, vibrates at its resonant frequency.
It is super small, smaller (~20 microns), in fact, than the thickness of human hair (~50 microns). Due to its size, it vibrates at a high frequency of around 4 MHz! Of course, you cannot hear this sound. But if you flow -say- a gold nanoparticle inside the tube, the nanoparticle will add its mass to the vibration of the whole tube. This addition will make our ‘miniature string’ minutely heavier, thus decreasing its resonant frequency by a couple of Hz.
We can measure this small change in resonant frequency and back-calculate the mass of the nanoparticle -in our case, the virus- that caused it. The more DNA is packed inside the virus, the greater its mass will be.
If you cannot hear this change of frequency, how do you measure it then?
You do what movie villains do. Use lasers! We shoot a laser light beam on the vibrating tube and track its reflection using photosensors. Light is pretty fast; using this approach, we can measure the vibration frequency even at this high range of MHz.
What aspect of this research did you find the most exciting?
The most exciting aspect was coming up with solutions to make the measurement work. On paper, the measurement looked pretty straightforward. In reality, the path to success is never pretty, straight, or forward! But that’s exactly where the beauty of science lies.
What challenges did you then have to overcome during the research?
Our virus (AAV) is incredibly small (~20 nanometers), which is a thousand times smaller than human hair.
When we flowed a single AAV in the nanomechanical resonator, it produced a signal that was hidden behind the experimental noise. To circumvent this problem, we flowed multiple viruses in the resonator only to obtain a complex signal, distinct from noise but still one that we did not understand.
To understand our signal, we had to write down an analytical theory and perform stochastic simulations to capture the physics at the nanoscale. Eventually, we had to develop a “denoising” method to eliminate the effects of omnipresent noise before successfully measuring the DNA cargo of our viruses. In fact, we even used gold nanoparticles to validate our measurement before moving on with viruses.
What properties of gold nanoparticles made them suited to this project?
Viruses are complex biological materials often of unknown or difficult to characterize composition. Contrary to viruses, gold nanoparticles can be fabricated with known size and composition. Thus, we used these nanoparticles as reference standards for validating the mass measurement. In particular, we first took 5nm-diameter gold nanoparticles, which have roughly the same mass as our viruses, and measured their mass, which was consistent with the expected reference value.
Compared to viruses like AAV, which have enormous therapeutic potential, gold nanoparticles are mundane materials. Yet, they provided that fundamental ‘check’ for our method. To take some liberty quoting one of my inspirations ‘The Last Lecture’ by Randy Pausch, "you have to work out the fundamentals before the fancy stuff works."
A key aspect of the system was that AAV measurements could take place in aqueous solutions. How did you approach this?
AAV are produced in aqueous solutions, which are routinely filtered using chromatography methods before we perform characterization. We did not need to resuspend AAV to a different solution. Our system measures AAV requiring a minimal amount of sample (1-5 μL). As a flow-through method, it could be integrated with manufacturing units for the characterization of AAV products along the production line.
Why are real-time quality control strategies critical for the mass production of AAV gene therapies?
Let me answer this from a historical perspective; more than a century ago, Ford’s assembly line enabled continuous, mass production of cars. Today we are interested in a different assembly line of gene therapy products such as AAV. But unlike Ford’s assembly line, where a foreman could easily walk down the production line, assess the quality of various parts and make decisions on the fly, employing real-time quality control in a biomanufacturing assembly line is challenging.
Traditional quality controls, such as PCR and ELISA are laborious and slow; by the time you obtain an answer, you might already have produced a lot of useless gene therapy product. Real-time quality control may allow optimizing the production line while it is running, boosting the production of high-quality products.
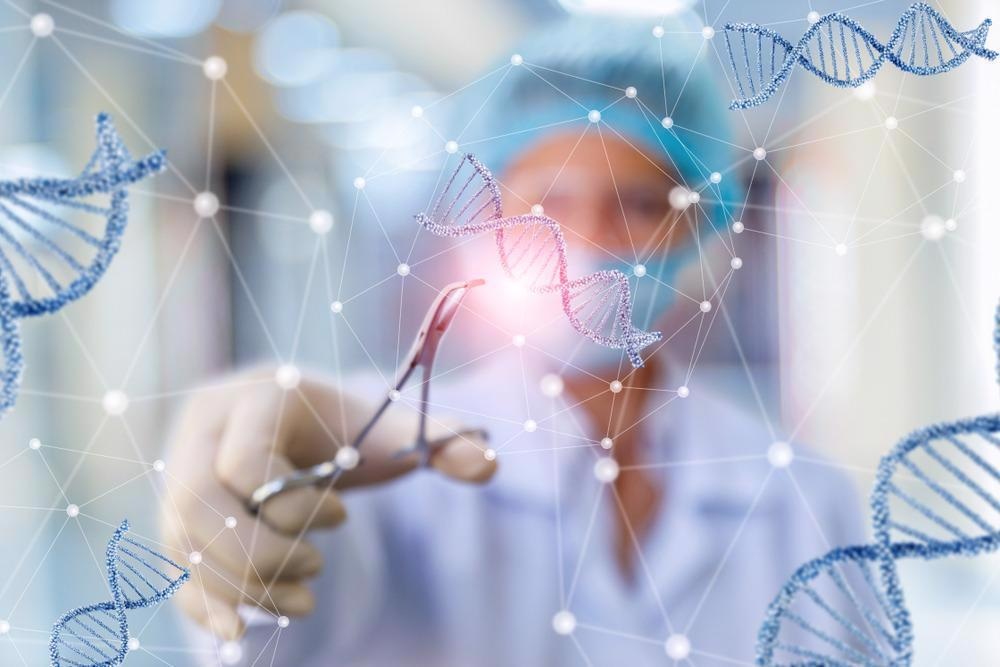
© Natali _ Mis/Shutterstock.com
We have seen nanotechnologies become increasingly integrated into the field of biomedicine, though attention tends to be on drug delivery vehicles or antibiotic agents. How can nanotechnologies benefit gene therapy?
Nanotechnologies benefit gene therapy by enabling new ways of manufacturing drug delivery vehicles and providing quality control during or post-manufacturing. In fact, before this research, we had reviewed the current state-of-the-art of these technologies in the context of AAV-based gene therapy.
What are the main barriers that would limit the integration of this system into standard practice?
Like other technologies for characterizing AAV, our system has its own strengths and limitations. It can quantify not only the average DNA content in a solution of AAV, but also the degree of aggregation.
However, it is a sensitive device; its nanochannels are prone to clogging if the sample is not purified. Furthermore, it has a considerable cost as it needs to be fabricated in a clean room setting. Translating it from academia to a biopharma setting would require work interfacing it with other instruments as well.
What are the next steps for this project, and where can our readers go to stay up to date with its progression?
In addition to the nanomechanical resonators, we plan to use micromechanical resonators large enough to measure biophysical changes in re-engineered cells that produce AAV. Our hypothesis is that identifying such changes in virus-producing cells could serve as a rapid proxy for the efficiency of viral production itself, as opposed to existing time-consuming viral potency assays.
I want to stress that this project is part of a three-pronged research thrust funded by the U.S. Food and Drug Administration, and the Massachusetts Life Science Center. We plan to juxtapose our new characterization technologies with molecular engineering and mathematical modeling of AAV production process, performed by my collaborators here at MIT.
I invite you to visit the Center of Biomedical Innovation here at MIT which coordinates efforts between different departments at MIT and biopharma partners.
About Dr. Georgios Katsikis
Georgios 'Yorgos' Katsikis is a postdoctoral associate at MIT. His research focuses on analytical technologies for characterizing viral vectors. Prior to MIT, he worked as an R&D engineer at Carbon, a 3D printing startup company in Silicon Valley.
He earned his Ph.D. in mechanical engineering from Stanford University. Before this, he completed his undergraduate studies in Mechanical Engineering at the National Technical University of Athens in Greece. His research interests include microfluidics, biomedical devices, biophysics, and rapid prototyping.
Disclaimer: The views expressed here are those of the interviewee and do not necessarily represent the views of AZoM.com Limited (T/A) AZoNetwork, the owner and operator of this website. This disclaimer forms part of the Terms and Conditions of use of this website.