Spintronics is a fast-growing new field in science and technology that has the potential to make a significant impact on all future electronics in the next few decades. This article explores the history and applications of the field, and touches on its even more promising future.
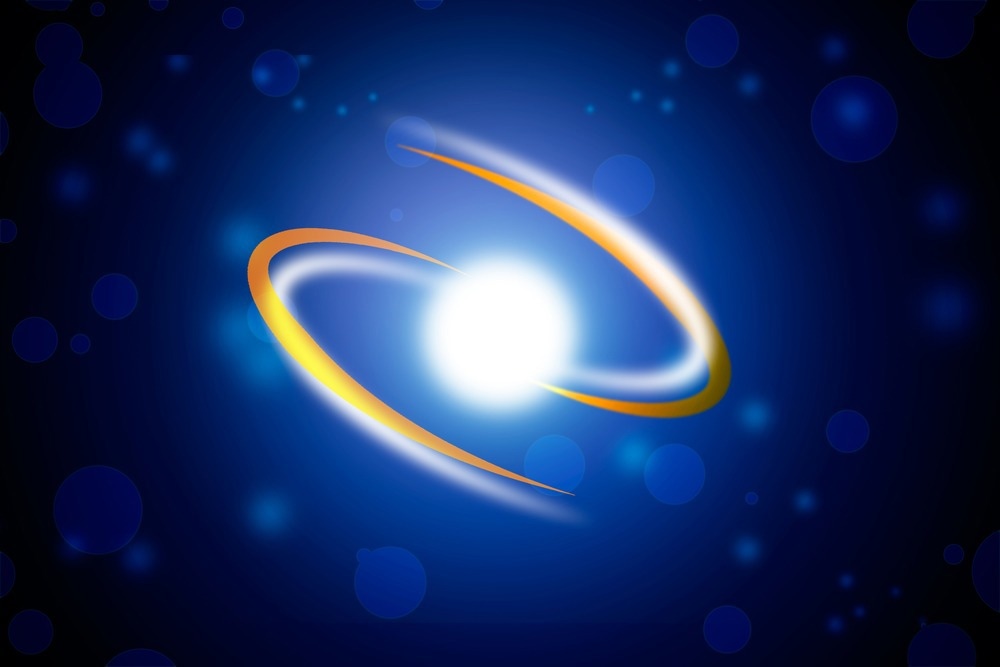
Image Credit: marie_mi/Shutterstock.com
What is Spintronics?
Spintronics is a fundamentally different approach to building programmable systems using electricity. As well as exploiting electrons’ charge states as in the case of traditional electronics, spintronics also makes use of electrons’ spin states.
Adding this second degree of freedom has significant implications for data storage and transfer applications, potentially increasing performance and efficiency over current technology by multiple factors.
Applications include dilute magnetic semiconductors (DMS), Heusler alloys, quantum computers, neuromorphic computing, and memory.
Spintronic systems are most often realized in dilute magnetic semiconductors (DMS) and Heusler alloys and are of particular interest in the field of quantum computing and neuromorphic computing.
A History of Spintronics
Spintronics is concerned with the intrinsic spin state of electrons in solid state devices, the magnetic moment associated with this, and the electrons’ charge.
The field has its origins in the 1960s, when IBM researchers led by Japanese physicist Leo Esaki discovered spin transfer electronic effects and their potential. Esaki won the Nobel Prize in 1973 for his contribution to the discovery of electronic tunneling.
Esaki’s team studied magnetoresistance in the antiferromagnetic barriers of Europium selenide (EuSe) between layers of metal electrodes. Since then, semiconductor thin film deposition techniques like molecular beam epitaxy have been advanced to the point where scientists could study magnetic multilayers.
In the 1970s and 1980s, a cluster of discoveries about electron spin transport phenomena in solid state devices received interest from scientists worldwide.
This research includes the original magnetic tunnel junction experiments by Michel Jullière in the 1970s, as well as Robert Meservey and Paul Tedrow’s early ferromagnet and superconductor experiments around the same time.
In the 1980s, Mark Johnson and R.H. Silsbee’s observation of spin polarized electron injection between two metals (one ferromagnetic metal and one nonmagnetic metal) in 1985, and Albert Ferd and Peter Grünberg’s simultaneous discovery of giant magnetoresistance (GMR) in 1988 brought spintronics bursting into maturity.
The discovery of GMR eventually led to the development of powerful new magnetic sensors. In turn, the development of magnetic sensors increased the information density capacity of hard disk drives and led to Albert Fert and Peter Grünberg sharing the 2007 Nobel Prize in Physics.
In the 1990s, semiconductor materials began to be used for spintronics after Supriyo Datta and Biswajit Das made the first attempt at a theoretical proposal for a spin field-effect transistor device.
In 1996, the term spintronics – an abbreviation of spin transfer electronics – was coined by the US government’s Defense Advanced Research Projects Agency (DARPA) as the name for a new program of research.
The DARPA program was initially concerned with advanced magnetic memory and spin transport electronic-based sensors. It was subsequently expanded in scope to include spin states in semiconductors’ electrons to develop new semiconductor electronics that exploit the extra spin degree of electron freedom.
Spintronic Memory
In the early 2000s, spin polarized transport in bulk and low dimensional semiconductor structures was examined, and the results of these studies implied that hybrid devices combining magnetic information gain and storage – spin memory transistors, in other words – were possible.
Magnetoresistive random access memory (MRAM) was duly introduced in 2006 with a relatively humble 4 Mb offering from advanced memory developer, FreeScale. In just a decade, capacity had grown to 256 Mb, with Everspin’s 2016 MRAM device.
To achieve this, MRAM technology has developed and transformed. Initially, a magnetic field based on switching technology was used to write data, but this technology would be impossible to scale below 100 nm in size.
However, the writing mechanism in newer MRAM devices is based on spin transfer torque (STT) and can be scaled to extremely low dimensions for nanotechnology applications.
The storage material has also seen improvements in this timeframe, with in-plane magnetic anisotropy materials eventually being replaced with materials that have perpendicular magnetic anisotropy. This switch makes MRAM scalable to higher densities.
What is Next in Spintronics?
The impact of spintronics is likely to increase further into the future.
MRAM will probably play an increasingly important role in advanced computer science, with the extra capacity for standalone memory sure to prove useful.
The widespread adoption of MRAM technology will depend on economic factors, however, and the future of advanced computing is uncertain. In terms of memory, advances in data transfer and data network capacity may lead cloud memory to make the need for excessive local memory obsolete in all but specialist applications.
Technological developments will also direct spintronics’ fate in the future. Scientists are finding out now if new materials, methods for controlling spin, and crystallinity in oxides will bring about the next step forward in the field of spintronics.
References and Further Reading
Baibich, N.M., et al. (1988). Giant Magnetoresistance of (001)Fe/(001)Cr Magnetic Superlattices. Physical Review Letters, 61 p. 2472. doi.org/10.1103/PhysRevLett.61.2472.
Bhatti, S., et al. (2017). Spintronics based random access memory: a review. Materials Today, 20(9), pp.530-548. doi.org/10.1016/j.mattod.2017.07.007.
Binasch, G., et al. (1989). Enhanced magnetoresistance in layered magnetic structures with antiferromagnetic interlayer exchange. Physical Review B, 39, 4828(R). doi.org/10.1103/PhysRevB.39.4828.
Datta, S., and B. Das (1990). Electronic analog of the electro‐optic modulator. Applied Physics Letters , 56, p. 665. doi.org/10.1063/1.102730.
Johnson, M., and R.H. Silsbee (1970). Interfacial charge-spin coupling: Injection and detection of spin magnetization in metals. Physical Review Letters, 55, p. 1790. doi.org/10.1103/PhysRevLett.55.1790.
Julliere, M. (1975). Tunneling between ferromagnetic films. Physics Letters A, 54(3), pp. 225-226. doi.org/10.1016/0375-9601(75)90174-7.
Ohno, H., et al. (2017). Advancements in Spintronics. [Online] NIST. Available at: https://www.nist.gov/publications/advancements-spintronics
Wolf, S.A., et al. (2006). Spintronics—A retrospective and perspective. IBM Journal of Research and Development, 50(1), pp. 101 - 110. doi.org/10.1147/rd.501.0101
Disclaimer: The views expressed here are those of the author expressed in their private capacity and do not necessarily represent the views of AZoM.com Limited T/A AZoNetwork the owner and operator of this website. This disclaimer forms part of the Terms and conditions of use of this website.