A study published in the journal PNAS reports the development of nanosized and metastable molybdenum oxide as efficient negative electrode material for aqueous electrolytes in lithium-ion batteries. High charge density, capacity, and stability are key findings.
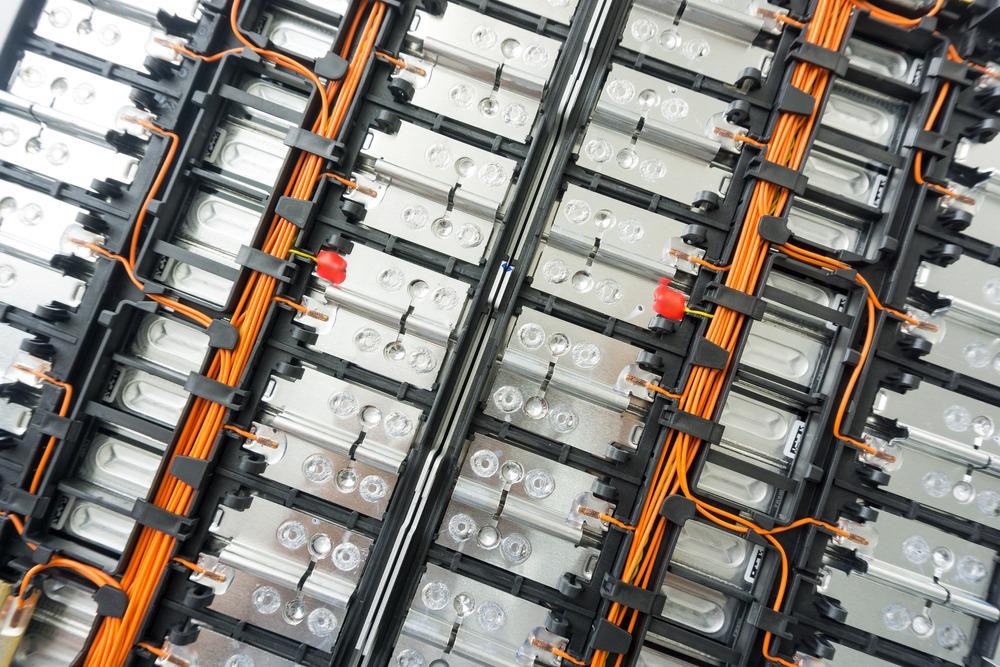
Study: Nanosized and metastable molybdenum oxides as negative electrode materials for durable high-energy aqueous Li-ion batteries. Image Credit: Smile Fight/Shutterstock.com
Rechargeable batteries are in huge demand due to the popularity of using portable devices like mobile phones and laptops, to electric vehicles.
Generally, a battery consists of two electrodes, an anode (the reductant) and a cathode (the oxidant), that is separated by an electrolyte that transfers the ionic component of chemical reaction inside the cell. The output of the battery is current at a specific voltage for the time duration depending on the charge stored.
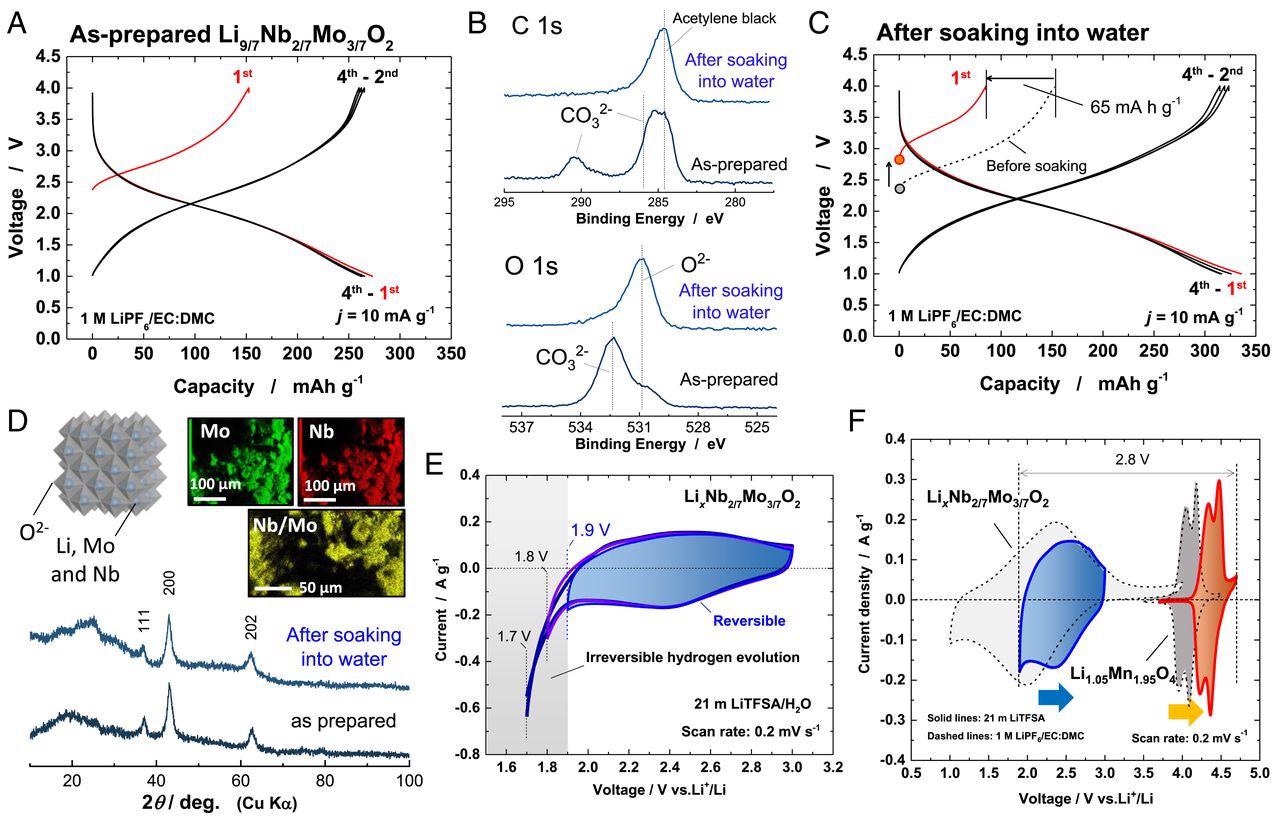
Characterization of LixNb2/7Mo3/7O2. (A) Charge/discharge curves (in a nonaqueous cell) of as-prepared Li9/7Nb2/7Mo3/7O2. (B) SOXPES spectra of C 1s and O 1s core levels of the sample before and after soaking in water. (C) Charge/discharge curves of LixNb2/7Mo3/7O2 after soaking in water. (D) X-ray diffraction (XRD) patterns of the sample before and after soaking in water and energy-dispersive X-ray spectroscopy (EDX) elemental maps of the sample after soaking in water. A schematic illustration of the crystal structure of LixNb2/7Mo3/7O2 drawn using the program VESTA (33) is also shown. (E) Cyclic voltammograms of LixNb2/7Mo3/7O2 in 21 m LiTFSA at a scan rate of 0.2 mV ⋅ s−1. A blue vertical line shows the lowest potential limit available in 21 m LiTFSA aqueous electrolyte. (F) Cyclic voltammograms of Li1.05Mn1.95O4 and LixNb2/7Mo3/7O2 in 21 m LiTFSA (solid lines) and 1 M LiPF6/EC:DMC (dashed lines), respectively. Image Credit: Suo, L., et al.
Lithium Ion Batteries
Currently, lithium-ion batteries (LIBs) are considered best among rechargeable batteries due to their higher energy density and efficiency.
LIBs used for electric vehicles have 50 kWh battery power, which can provide energy for a 300 km drive. Moreover, grid-scale energy storage systems require battery power on a scale of megawatt-hour to gigawatt-hour.
However, due to the use of flammable organic electrolytes, large-scale production of LIBs raises safety issues. A potential method of resolving these issues involves the use of aqueous electrolytes, which have other benefits such as higher ionic conductivity and environmental safety.
The use of aqueous electrolytes results in few demerits in LIBs compared to organic electrolytes, one of them is the lower energy density due to low operating voltage of LIBs with aqueous electrolytes.
This condition arises due to the slow kinetics of water electrolysis resulting in a narrow electrochemical stability window of aqueous electrolytes. The operation voltage window of aqueous electrolytes is typically <1.8 V.
There are many options for the cathode material that could work in the stability window of the aqueous electrolytes; however, the choice of negative electrolyte is limited. In addition, conventional anode materials exhibit hydrogen evolution reactions.
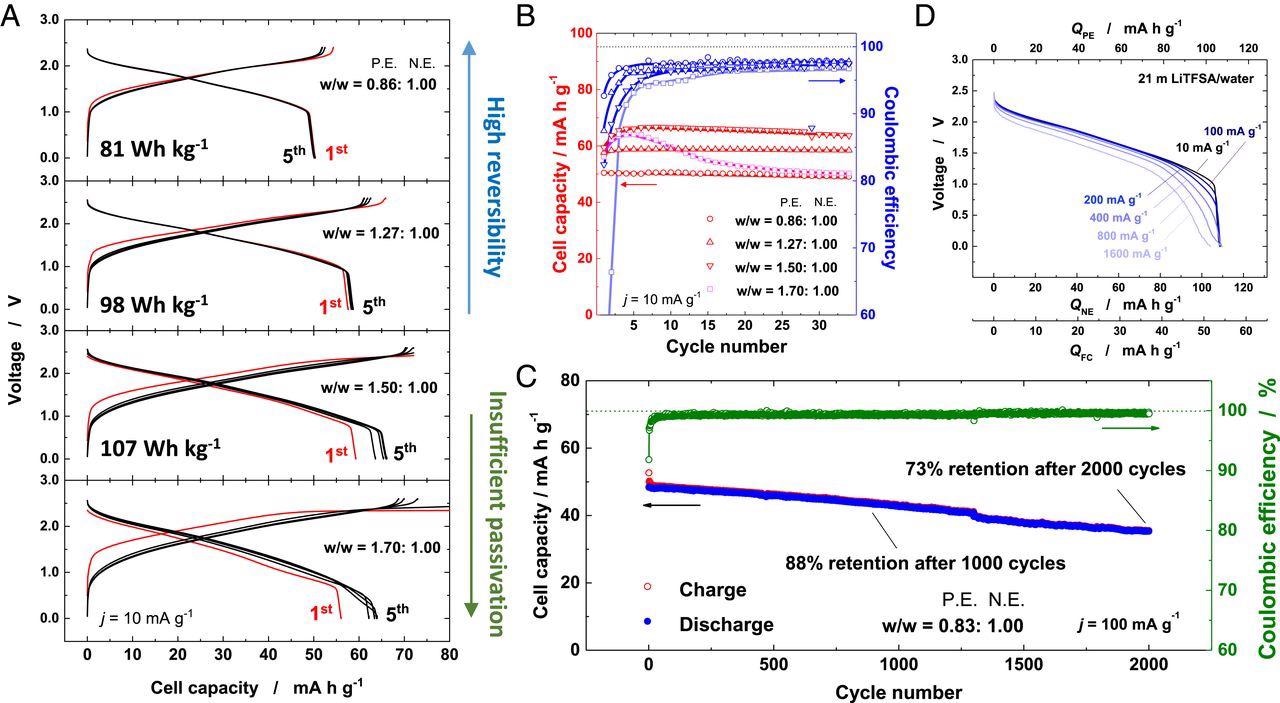
Electrochemical properties of Li1.05Mn1.95O4/LixNb2/7Mo3/7O2 full cells. (A) Comparison of charge/discharge curves of Li1.05Mn1.95O4/LixNb2/7Mo3/7O2 full cells consisting of different weight ratios of positive electrodes to negative electrodes at a rate of 10 mA ⋅ g−1 and (B) their capacity retention and Coulombic efficiency for 35 cycles in 21 m LiTFSA/H2O. (C) Long-term cycling stability performance of the full cell for 2,000 cycles at a rate of 100 mA ⋅ g−1 and (D) rate capability of the full cell in 21 m LiTFSA/H2O. Image Credit: Suo, L., et al.
Water in Salt Electrolytes for Aqueous LIBs
In 2015, Suo et al. introduced the “water in salt” electrolytes that exhibited a wider stability window of ~3 V. Saturated 21 M lithium bis (trifluoromethanesulfonyl) amide (LiTFSA) aqueous electrolytes being one of its examples. A reduction in water concentration resulted in suppression of oxygen evolution reaction that led to higher decomposition potential upon oxidation.
A full cell of LiMn2O4/Mo6S8 in 21 M LiFTSA aqueous electrolyte reported an energy density of 84 Wh.kg-1 at a 0.2-C rate. The latest study reports the energy density of 130 Wh.kg-1 for aqueous LIBs consisting of Li4Ti5O12 as negative electrode material.
However, due to the unavoidable and simultaneous decomposition reaction of water molecules upon electrochemical cycles, high energy density was achieved only at higher rates. Moreover, the high rates of charge and discharge limited the utilization of negative electrode capacity to ~100 mA.h.g-1.
Nanosized and Metastable Molybdenum Oxide as Negative Electrode Material
The present study by Yun et al., reports a new negative electrode material that exhibits high capacity and high durability in aqueous LIBs. The electrode of lithium excess molybdenum oxide containing niobium ions, Li9/7Nb2/7Mo3/7O2, was synthesized through mechanical milling of LiMoO2 and Li3NbO4. Mechanical milling was earlier proved to synthesize efficient metastable materials.
Due to the oxidation of the material upon contact with moisture, defect sites in the material bulk and the presence of LiOH at the surface of oxide particles were reported. The surface chemical analysis of the material before and after soaking in water was studied and further analyzed.
The material exhibited the presence of Li2CO3 before soaking in water, indicating the adsorption of CO2 gas by LiOH. Analysis of material after soaking in water showed the removal of LiCO3 and further oxidation of molybdenum oxides.
This oxidation of molybdenum oxide was reported beneficial when used as negative electrode material. The LiCO3 becomes dissolved in water, and a cleaner surface of oxide particles is reported.
However, no significant change in the crystal structure, even after soaking in water, was reported. The material retained cation disordered rock salt structure after soaking in water with a slight change in lattice parameters.
Energy-dispersive X-ray elemental mapping elucidated the uniform distribution of Nd and Mo ions in the material, which indicated that the Li ions were extracted topotactically from the oxides.
LixNd2/7Mo3/7O2 (Li excess metastable state) electrode material was examined for electrode performance and stability through cyclic voltammetry (CV) in an aqueous solution of 21 M LiTFSA electrolyte. The results exhibited high capacity and long cycle life stability for aqueous systems.
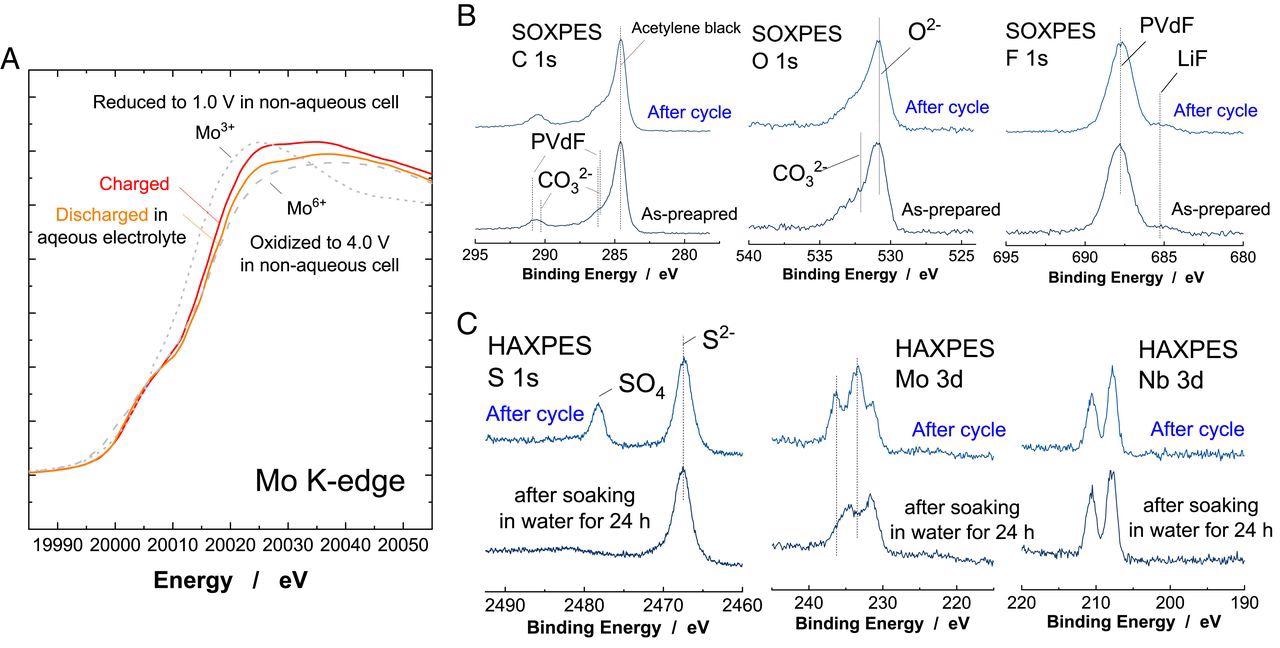
Characterization of LixNb2/7Mo3/7O2 cycled in the aqueous electrolyte. (A) Changes in Mo K-edge XAS spectra of LixNb2/7Mo3/7O2 after cycle in the aqueous electrolyte (the mass loading ratio of the positive electrode to negative electrode was set to 1.0). The data collected in nonaqueous electrolyte is also shown for comparison. (B) SOXPES spectra of the composite LixNb2/7Mo3/7O2 electrodes before and after cycle in 21 m LiTFSA/H2O. The full cell was cycled in the range of 0 to 2.6 V for five cycles at a rate of 10 mA ⋅ g−1 (the mass loading ratio; 1.5), and then the negative electrode was taken out from the cell for the measurement. (C) HAXPES spectra of the cycled electrode after rinse by water for a short time (denoted as “after cycle”) and after soaking in water for 24 h. Other data sets are found in SI Appendix, Fig. S12. Image Credit: Suo, L., et al.
A protective passivation layer over LixNd2/7Mo3/7O2 was observed after cycling in 21 M LiTFSA, which exhibited effective suppression of hydrogen evolution reaction. This surface layer was formed by the sacrificial decomposition of electrolyte used, which further enhanced the available capacity of LixNd2/7Mo3/7O2.
The optimized aqueous LIBs exhibited a high energy density of 107 Wh.kg-1, even at a slow rate. In addition, high durability of ~73 % of capacity retention for over 2,000 cycles at 100 mA.g-1 was reported with a full cell.
Future Scope of the Study
This study reported LixNd2/7Mo3/7O2 as an efficient negative electrode material for aqueous lithium-ion batteries, exhibiting high charge densities and capacity. Results contribute to the development of safe and durable aqueous LIBs, and indicate the scope of material development for enhanced performances of aqueous lithium-ion batteries.
Reference
Yun, J., Sagehashi, R., Sato, Y., Masuda, T., Hoshino, S., Rajendra, H.B., Okuno, K., Hosoe, A., Bandarenka, A.S. and Yabuuchi, N. (2021) Nanosized and metastable molybdenum oxides as negative electrode materials for durable high-energy aqueous Li-ion batteries. Proceedings of the National Academy of Sciences, 118 (48). Available at:https://nanoscalereslett.springeropen.com/articles/10.1186/s11671-021-03613-z
Further Reading
Suo, L., Borodin, O., Gao, T., Olguin, M., Ho, J., Fan, X., Luo, C., Wang, C. and Xu, K. (2015) “Water-in-salt” electrolyte enables high-voltage aqueous lithium-ion chemistries. Science, 350 (6263), pp.938-943. Available at: https://doi.org/10.1126/science.aab1595.
Goodenough, J.B. and Park, K.S. (2013) The Li-ion rechargeable battery: a perspective. Journal of the American Chemical Society, 135 (4), pp.1167-1176. Available at: https://doi.org/10.1021/ja3091438.
Disclaimer: The views expressed here are those of the author expressed in their private capacity and do not necessarily represent the views of AZoM.com Limited T/A AZoNetwork the owner and operator of this website. This disclaimer forms part of the Terms and conditions of use of this website.