Silver nanoparticles have unique optical, electrical, and thermal properties and are being incorporated into products that range from photovoltaics to biological and chemical sensors. Examples include conductive inks, pastes and fillers which utilize silver nanoparticles for their high electrical conductivity, stability, and low sintering temperatures. Additional applications include molecular diagnostics and photonic devices, which take advantage of the novel optical properties of these nanomaterials.
An increasingly common application is the use of silver nanoparticles for antimicrobial coatings, and many textiles, keyboards, wound dressings, and biomedical devices now contain silver nanoparticles that continuously release a low level of silver ions to provide protection against bacteria.
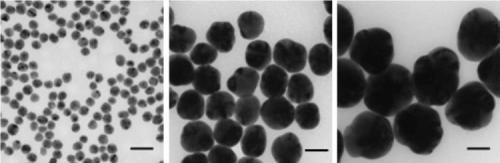
Figure 1. Transmission electron microscopy (TEM) images of silver nanoparticles with diameters of 20 nm (Aldrich Prod. No. 730793), 60 nm (Aldrich Prod. No. 730815), and 100 nm (Aldrich Prod. No. 730777) respectively. Scale bars are 50 nm.
Understanding how the size, shape, surface, and aggregation state of the silver nanoparticles change after integration into a target application is critical for optimizing performance. Aldrich Materials Science offers precisely manufactured monodisperse silver nanoparticles that are free from agglomeration, making them ideal for research, development, and use in a variety of innovative applications (Figure 1).
Each batch of nanoparticles is extensively characterized using transmission electron microscopy (TEM) images, dynamic light scattering (DLS, for particle size analysis), Zeta potential measurements, and UV/Visible spectral analysis to ensure consistent materials. A list of silver nanoparticle dispersions available from Aldrich Materials Science is presented in Table 1.
Table 1. Silver Nanoparticle Dispersions
Product Number |
Nanoparticle Diameter
(nm)
|
Mass Concentration
(mg/mL) |
Number concentration
(nanoparticles/mL) |
730785 |
10 nm |
0.02 mg/mL |
3.6x1012 |
730793 |
20 nm |
0.02 mg/mL |
4.5x1011 |
730807 |
40 nm |
0.02 mg/mL |
5.7x1010 |
730815 |
60 nm |
0.02 mg/mL |
1.7x1010 |
730777 |
100 nm |
0.02 mg/mL |
3.6x109 |
Silver Nanoparticle Optical Properties
There is growing interest in utilizing the optical properties of silver nanoparticles as the functional component in various products and sensors. Silver nanoparticles are extraordinarily efficient at absorbing and scattering light and, unlike many dyes and pigments, have a color that depends upon the size and the shape of the particle. The strong interaction of the silver nanoparticles with light occurs because the conduction electrons on the metal surface undergo a collective oscillation when excited by light at specific wavelengths (Figure 2, left).
Known as a surface plasmon resonance (SPR), this oscillation results in unusually strong scattering and absorption properties. In fact, silver nanoparticles can have effective extinction (scattering + absorption) cross sections up to ten times larger than their physical cross section. The strong scattering cross section allows for sub 100 nm nanoparticles to be easily visualized with a conventional microscope. When 60 nm silver nanoparticles are illuminated with white light they appear as bright blue point source scatterers under a dark field microscope (Figure 2, right).
The bright blue color is due to an SPR that is peaked at a 450 nm wavelength. A unique property of spherical silver nanoparticles is that this SPR peak wavelength can be tuned from 400 nm (violet light) to 530 nm (green light) by changing the particle size and the local refractive index near the particle surface. Even larger shifts of the SPR peak wavelength out into the infrared region of the electromagnetic spectrum can be achieved by producing silver nanoparticles with rod or plate shapes.
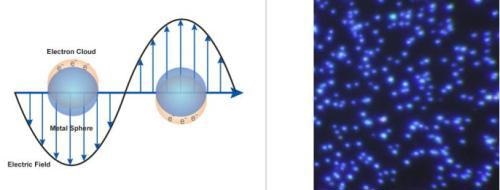
Figure 2. (Left) Surface plasmon resonance where the free electrons in the metal nanoparticle are driven into oscillation due to a strong coupling with a specific wavelength of incident light. (Right) Dark field microscopy image of 60 nm silver nanoparticles (Aldrich Prod. No. 730815).
Silver Nanoparticle Characterization
The size and shape of metal nanoparticles are typically measured by analytical techniques such as TEM, scanning electron microscopy (SEM) or atomic force microscopy (AFM). Measuring the aggregation state of the particles requires a technique to measure the effective size of the particles in solution such as dynamic light scattering (DLS) or analytical disc centrifugation. However, due to the unique optical properties of silver nanoparticles, a great deal of information about the physical state of the nanoparticles can be obtained by analyzing the spectral properties of silver nanoparticles in solution.
The spectral response of silver nanoparticles as a function of diameter is shown in Figure 3, left. As the diameter increases, the peak plasmon resonance shifts to longer wavelengths and broadens. At diameters greater than 80 nm, a second peak becomes visible at a shorter wavelength than the primary peak. This secondary peak is due to a quadrupole resonance that has a different electron oscillation pattern than the primary dipole resonance. The peak wavelength, the peak width, and the effect of secondary resonances yield a unique spectral fingerprint for a plasmonic nanoparticle with a specific size and shape.
Additionally, UV-Visible spectroscopy provides a mechanism to monitor how the nanoparticles change over time. When silver nanoparticles aggregate, the metal particles become electronically coupled and this coupled system has a different SPR than the individual particles. For the case of a multi-nanoparticle aggregate, the plasmon resonance will be red-shifted to a longer wavelength than the resonance of an individual nanoparticle, and aggregation is observable as an intensity increase in the red/infrared region of the spectrum.
This effect can be observed in Figure 3, right, which displays the optical response of a silver nanoparticle solution destabilized by the addition of saline. Carefully monitoring the UV-Visible spectrum of the silver nanoparticles with time is a sensitive technique used in determining if any nanoparticle aggregation has occurred.
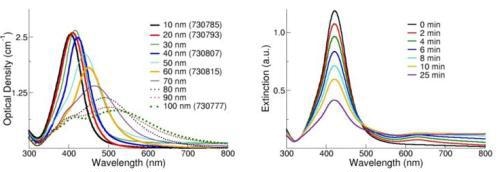
Figure 3. (Left) Extinction (scattering + absorption) spectra of silver nanoparticles with diameters ranging from 10-100 nm at mass concentrations of 0.02 mg/mL. (Right) Extinction spectra of silver nanoparticles after the addition of a destabilizing salt solution.
For silver nanoparticle solutions that have not agglomerated and have a spectral shape that is identical to the as- received suspension, the UV/Visible extinction spectra can be used to quantify the nanoparticle concentration.
The concentration of silver nanoparticle solutions is calculated using the Beer-Lambert law, which correlates the optical density (OD, a measure of the amount of light transmitted through a solution) with concentration. Due to the linear relationship between OD and concentration, these values can be used to quantify the concentration of nanoparticle solutions.
Silver Nanoparticle Surface Chemistry
When nanoparticles are in solution, molecules associate with the nanoparticle surface to establish a double layer of charge that stabilizes the particles and prevents aggregation. Aldrich Materials Science offers several silver nanoparticles suspended in a dilute aqueous citrate buffer, which weakly associates with the nanoparticle surface.
This citrate-based agent was selected because the weakly bound capping agent provides long term stability and is readily displaced by various other molecules including thiols, amines, polymers, antibodies, and proteins.
Silver Nanoparticle Applications
Silver nanoparticles are being used in numerous technologies and incorporated into a wide array of consumer products that take advantage of their desirable optical, conductive, and antibacterial properties.
- Diagnostic Applications: Silver nanoparticles are used in biosensors and numerous assays where the silver nanoparticle materials can be used as biological tags for quantitative detection.
- Antibacterial Applications: Silver nanoparticles are incorporated in apparel, footwear, paints, wound dressings, appliances, cosmetics, and plastics for their antibacterial properties.
- Conductive Applications: Silver nanoparticles are used in conductive inks and integrated into composites to enhance thermal and electrical conductivity.
- Optical Applications: Silver nanoparticles are used to efficiently harvest light and for enhanced optical spectroscopies including metal-enhanced fluorescence (MEF) and surface-enhanced Raman scattering (SERS).
Silver Nanoparticles for Nanotoxicology Research
There is growing interest in understanding the relationship between the physical and chemical properties of nanomaterials and their potential risk to the environment and human health. The availability of panels of nanoparticles where the size, shape, and surface of the nanoparticles are precisely controlled allows for the better correlation of nanoparticle properties to their toxicological effects.
Sets of monodisperse, unaggregated, nanoparticles with precisely defined physical and chemical characteristics provide researchers with materials that can be used to understand how nanoparticles interact with biological systems and the environment.
Due to the increasing prevalence of silver nanoparticles in consumer products, there is a large international effort underway to verify silver nanoparticle safety and to understand the mechanism of action for antimicrobial effects. Colloidal silver has been consumed for decades for its perceived health benefits1 but detailed studies on its effect on the environment have just begun. Initial studies have demonstrated that effects on cells and microbes are primarily due to a low level of silver ion release from the nanoparticle surface.
The ion release rate is a function of the nanoparticle size (smaller particles have a faster release rate), the temperature (higher temperatures accelerate dissolution), and exposure to oxygen, sulfur, and light. In all studies to date, silver nanoparticle toxicity is much less than the equivalent mass loading of silver salts.

This information has been sourced, reviewed and adapted from materials provided by Sigma Aldrich.
For more information on this source, please visit Sigma Aldrich.
Disclaimer: The views expressed here are those of the author expressed in their private capacity and do not necessarily represent the views of AZoM.com Limited T/A AZoNetwork the owner and operator of this website. This disclaimer forms part of the Terms and conditions of use of this website.