.jpg)
Shutterstock | foxaon1987
Quantum computing, which is a new paradigm in computing, utilizes the benefits of quantum mechanics to improve computing experience. Rather than relying on binary digits (0 and 1), which the computers have depended on since the early years, quantum computers will use quantum bits that can be in a superposition of states.
Quantum bits, or qubits, are able to exist in many states at the same time, providing parallel computing advantages. For instance, they have long been considered to be far superior to classical computers for applications in data encryption.
The concept of quantum computers has been known for many years, yet practical realizations are still lacking. The key limitation has been the critical influence of the surroundings on a qubit. Majority of physical systems need to be in impeccably controlled conditions in order to remain in the superposition state, while any interaction (mechanical, thermal, or other) with the environment perturbs this state and destroys the qubit. This kind of perturbation is called “decoherence” and has plagued numerous potential qubit systems.
Graphene: Advantages and Disadvantages
Graphene, which has spurred research into numerous novel directions, is naturally also regarded as a candidate material host for qubits. For instance, in 2013, a research team from MIT learnt that graphene can be made into a topological insulator. This means that electrons with one spin direction move around the graphene edges clockwise, while electrons with the opposite spin move counter clockwise. The team made this happen by applying two magnetic fields: one parallel to the graphene sheet, which separates the two spin contributions, and another perpendicular to the graphene sheet, to make the electrons flow at sheet edges only.
As electron spin is inherently a quantum system that is in a superposition of states, it has long been considered a candidate qubit. In graphene, the spins move along the sheet edges robustly, and do not exhibit much decoherence. Moreover, the same research showed switching the spin selection on and off, a key feature of q-bit transistors. Yet, extreme conditions such as strong magnetic fields and temperatures near absolute zero are necessary for this effect in graphene, thus raising questions about its practical applicability.
.jpg)
Image: Graphene spin qubit, MIT.
New Kind of Quantum State
This year, the same group found a new kind of quantum state that appears when graphene is packed between two superconductors. In this case, the electrons in graphene, previously behaving as individual, scattering particles, instead pair up in “Andreev states” — a fundamental electronic configuration that enables a traditional, non-superconducting material to carry a “super-current,” an electric current that flows without dissipating energy. Similar to the spin qubits, Andreev states have very little decoherence owing to their paired configuration. These states are expected to give rise to Majorana fermions, exotic particles that can be used for quantum computing. Even though this experiment is also carried out at low temperatures, it is an important proof-of-concept that may pave the way towards practical realizations of quantum computing in the future.
Most recently, a group from EPFL in Switzerland developed a new way to use graphene in quantum electronics. In a layered capacitor structure, where boron nitride makes the insulating layer and graphene forms the capacitor parallel plates, quantum capacitance gives rise to novel nonlinear electronic phenomena. In this system, slight changes in, say, the intensity of an incident laser beam, lead to major changes in the measured capacitance of the device. The researchers calculate that one single incident photon may suffice to alter the qubit states, which is an ideal case of a qubit. Again, low temperatures are required for operation, but a major advantage of this design is that it does not require external magnetic fields, bringing this solution a step closer in the direction of practical applications.
Conclusion
To summarize, there are various proposals to use graphene in quantum computers. From spin qubits, to nonlinear capacitors, to Majorana fermions, each has their own advantage. One common theme is that all these solutions are highly novel and innovative, and that the combination of quantum computing and 2D materials is inevitable in the long run.
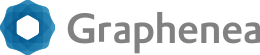
This information has been sourced, reviewed and adapted from materials provided by Graphenea.
For more information on this source, please visit Graphenea.