Vanadium redox flow batteries (VRFB) are among the most promising technological developments for the high-efficiency storage of renewable energy.
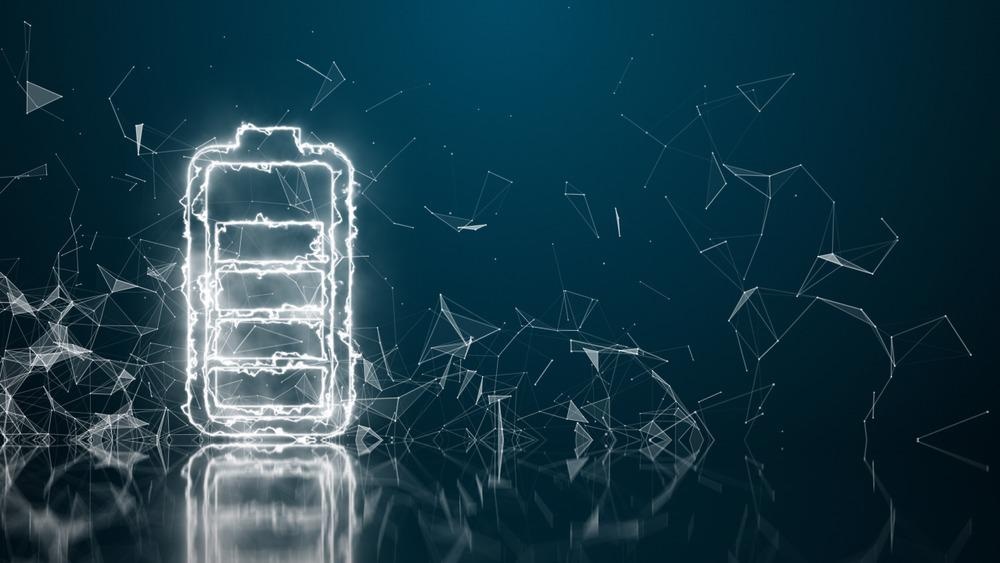
Image Credit: Immersion Imagery/Shutterstock.com
The most commonly used commercial ion-exchange membranes in VRFB, such as Nafion or perfluorinated sulfonic acid (PFSA), exhibit high vanadium ion permeation, which results in poor battery performance and cyclic stability. Recently, a group of researchers from the Shenzhen Institute of Advanced Technology in China developed a hybrid ion-exchange membrane using graphene-based nanomaterials, which dramatically improved the performance of the VRFBs.
With the ongoing trend toward using renewable energy sources for energy generation, the interest in advanced energy storage methods has risen. Solar and wind power generation rarely provide consistent and immediate delivery of electricity following the ever-fluctuating demand of the consumers. Storing renewable energy for subsequent delivery to the power grid in a controlled manner is currently the favored method by the power generation industry to resolve this issue.
How to Store Renewable Energy?
More than 90% of the grid storage of electricity globally employs the pumped hydroelectric storage method. Here, the energy is stored as the potential energy of water in an elevated reservoir (pumped from a lower elevation reservoir). Such energy storage solutions require expensive infrastructure and are often limited by geographical and environmental factors.
Finding a suitable and scalable energy storage method capable of enduring a high number of charging/discharging cycles without losing capacity is crucial for harnessing the power of renewable energy sources.
Conventional battery storage is the second preferred energy storage method accounting for around 5% of the global grid electricity storage. However, conventional batteries have major drawbacks - the cheaper and heavier lead-acid technology can only withstand a limited number of cycles before losing storage capacity, while the lithium-based technology proves too expensive for large-scale applications.
Redox Flow Batteries as a Superior Alternative to Conventional Batteries
Emerging techniques for energy storage, such as the VRFB, promise to resolve some of these issues. Unlike conventional batteries, where the electrolyte is sealed within the battery cell, the redox flow batteries utilize externally stored liquid electrolyte delivered to the battery whenever required to generate electricity.
Importantly, this concept ensures that the power output and storage capacity of the VRFB are independent since the output power depends on the electrolyte flow rate and configuration of the cell stack, whereas the battery capacity depends solely on the amount of stored electrolyte.
The Working Principle of the VRFBs
Among the various available flow battery technologies, the VRFBs are the most reliable as they use the same metal ions (vanadium) in the two liquids electrolytes used in the battery, thus alleviating possible electrolyte cross-contamination problems when compared to flow batteries that use dissimilar electrolytes.
The VRFBs use vanadium ions in four different oxidation states - V2+, V3+ ions stored in the negative electrolyte, and VO2+ and VO2+ ions stored in the positive electrolyte. Within the battery pack, the two types of electrolytes are separated by an ion-exchange membrane that permits a selective ion exchange while preventing the cross-contamination of electrolytes and cathode/anode short circuit.
Currently, Nafion and other PFSA-based ion-exchange membranes are the most widely used in VRFBs. However, these membranes exhibit considerable vanadium ion permeation, which leads to a shorter lifetime and unsatisfactory performance of the VRFB.
Two-Dimensional Nanosheets as an Efficient Barrier
Now, a research group from the Shenzhen Institute of Advanced Technology in China developed a hybrid ion-exchange membrane, where graphene oxide (GO) nanosheets were embedded in the PFSA matrix to act as a barrier for the migrating vanadium ions.
Due to its chemical stability and large specific surface area, GO is a promising material that can be used to modify the morphology and properties of ion-exchange membranes. However, because of the strong electrostatic interaction between the GO nanosheets, even at concentrations as low as 0.01 wt% the nanosheets aggregate during the polymer membrane fabrication, thus degrading the stability of such hybrid membranes.
To overcome the aggregation problem, the Shenzhen research team led by Prof. Huiyun Li used tungsten trioxide (WO3) nanoparticles in-situ grown on the surface of the GO nanosheets. The nanoparticles screened the electrostatic attraction between the GO nanosheets while enhancing their hydrophilicity and dispersibility within the polymer matrix. To improve the mechanical properties of the hybrid ion-exchange membrane, the researchers embedded a thin porous layer of polytetrafluoroethylene (PTFE) in the middle of the PFSA-GO-WO3 hybrid structure to act as a reinforcement layer.
Nanotechnology Improves the Performance of VRFB
When Prof. Li's team tested the electrochemical performance of the novel hybrid membrane, they discovered that the WO3 nanoparticles grown on the GO nanosheets acted as proton active sites that shortened the proton diffusion paths across the membrane. A direct comparison of the performance of two identical VRFB cells, one assembled with commercial Nafion ion-exchange membrane and the other with the newly-developed membrane, revealed that hybrid ion-exchange membrane delivered energy efficiency of 88.9%, compared to 81% for the Nafion membrane. At the same time, the capacity decay of the PFSA-GO-WO3 membrane after 600 charge/discharge cycles was only 0.07% per cycle, which is six times lower than that of the Nafion membrane (0.44% per cycle).
One-Dimensional Nanowires Boost Ion-Exchange Performance
In a separate study, Prof. Li and her team demonstrated a composite ion-exchange membrane consisting of a porous PTFE support sandwiched between PFSA layers with embedded one-dimensional functionalized silicon carbide nanowires. The nanowire-containing hybrid ion-exchange membrane showed equally superior performance when compared to commercially available ion-exchange membranes while reducing the permeation of vanadium ions even better than the PFSA-GO-WO3 membrane.
Prof. Li's research showcases an effective strategy for boosting the performance of VRFBs by using one- and two-dimensional nanomaterials that can be extended to other applications of ion-exchange membranes, such as water purification and fuel cells.
Continue reading: A Review of Graphene in Energy Storage Devices
References and Further Reading
Ye, J., et al. (2021) In Situ Grown Tungsten Trioxide Nanoparticles on Graphene Oxide Nanosheet to Regulate Ion Selectivity of Membrane for High Performance Vanadium Redox Flow Battery. Adv. Funct. Mater., 2109427. Available at: https://doi.org/10.1002/adfm.202109427
Ye, J., et al. (2022) Advanced hybrid membrane for vanadium redox flow battery created by polytetrafluoroethylene layer and functionalized silicon carbide nanowires. Chem. Eng. J., 427, 131413. Available at: https://doi.org/10.1016/j.cej.2021.131413
Nanowerk (2021) Novel ion exchange membrane improves performance of vanadium redox flow batteries [Online] www.nanowerk.com Available at: https://www.nanowerk.com/nanotechnology-news2/newsid=59110.php
Aramendia, I., et al. (2021) Vanadium Redox Flow Batteries: A Review Oriented to Fluid-Dynamic Optimization. Energies 14, 176. Available at: https://doi.org/10.3390/en14010176
Thiam, B. G. and Vaudreuil, S. (2021) Review - Recent Membranes for Vanadium Redox Flow Batteries. J. Electrochem. Soc., 168, 070553. Available at: https://iopscience.iop.org/article/10.1149/1945-7111/ac163c
Lourenssen, K., et al. (2019) Vanadium redox flow batteries: A comprehensive review. J. Energy Storage, 25, 100844. Available at: https://doi.org/10.1016/j.est.2019.100844
Cunha, Á., et al. (2015), Vanadium redox flow batteries: a technology review. Int. J. Energy Res., 39, 889– 918. Available at: https://doi.org/10.1002/er.3260
Disclaimer: The views expressed here are those of the author expressed in their private capacity and do not necessarily represent the views of AZoM.com Limited T/A AZoNetwork the owner and operator of this website. This disclaimer forms part of the Terms and conditions of use of this website.