The discovery of graphene has motivated scientists to explore a wide range of layered, atomically-thin 2D materials. One of the most astounding discoveries was that such monoatomic layers could be combined to create altogether new materials with unique electrical and magnetic properties that differ from the properties of the corresponding bulk materials.
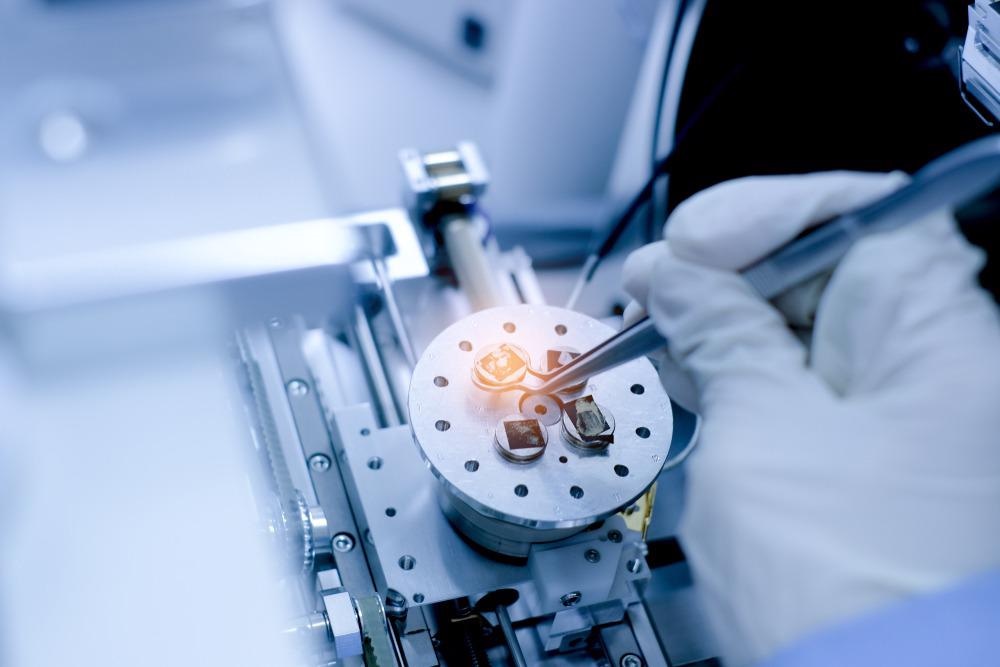
Image Credit: Anucha Cheechang/Shutterstock.com
Recent advances in material science uncovered the enormous potential of the 2D ferroelectric and ferromagnetic materials as building blocks for the next generation of low-power non-volatile memory.
Following the discovery of graphene in 2004, the scientific community has identified more than 2500 other atomically-thin 2D materials, such as hexagonal boron nitride, molybdenum disulfide, other dichalcogenides, and layered oxides. The various two-dimensional materials discovered so far offer a broad range of electronic properties, including insulators, semiconductors, semimetals, conductors, and superconductors.
Layered 2D Materials Uncover New Phenomena
While these materials exhibit extraordinary electrical, chemical, optical, and mechanical properties, perhaps one of the most groundbreaking discoveries was that atomically-thin layers of different materials can be combined freely to create even more unique and novel materials. Scientists have found that materials with different properties can emerge by stacking two or more layers of graphene-like materials.
The in-plane stability and order within the individual layers of the 2D materials are provided by strong covalent bonds. The stacked layers interact via weaker van der Waals forces that are sufficient to keep the stack together.
At the same time, the weaker inter-layer interactions allow the scientist to move and rotate the layers relative to each other and to tune the properties of the resultant hybrid 2D materials. The ability to assemble stacks of a wide range of different 2D materials with virtually no structural limitations paves the way for the design of new materials and nano-devices. The feasibility of creating multilayered van der Waals heterostructures was demonstrated experimentally in the early 2010s.
Pick-and-Mix at the Atomic Scale
Such stacks of different monoatomic layers act as a bulk material (within the individual layers) and an interface (the atomically-flat boundaries between the layers) simultaneously. These architectures offer a unique combination of rapid charge transport within the layers and the possibility to induce large electric potentials between the layers, thus opening opportunities for tunable band-gap and other optoelectronic properties of the van der Waals heterostructures.
One of the most versatile methods for creating the heterostructures is the mechanical transfer technique, where exfoliated flakes are stacked vertically using high-precision micromanipulators. This transfer technique proved to be very flexible and suitable for fabricating heterostructures by using a wide range of 2D materials.
Besides, intensive research efforts are focused on developing new synthetic methods that permit scalable synthesis of large-area heterostructures. Several vertical heterostructures have already been created by van der Waals epitaxy.
Ferroelectricity in Two Dimensions
A special subset of van der Waals heterostructures are the 2D ferroelectric materials that possess spontaneous electrical polarization without external electric fields. The polarization could be switched and controlled by applying a large enough external electric field. All ferroelectric materials only exhibit these properties below the Curie temperature (Tc), where a structural transition from high-symmetry to low-symmetry occurs.
Ferroelectricity was first discovered in the 1920s; however, a stable polarization in traditional ferroelectrics can only be maintained above a certain critical thickness of the material (approximately 10 nm). Otherwise, the electric dipoles in the materials spontaneously depolarize.
So far, a large number of 2D ferroelectric materials have been theoretically predicted, including transition metal thiophosphates (such as AgBiP2Se6, CuInP2S6, and CuInP2Se6), binary compounds combining elements from group IV or III–V (such as In2Se3), and other materials. In contrast, only a handful of 2D ferroelectric materials were confirmed experimentally.
In 2018 several researchers from the USA, UK, and China reported low-temperature ferroelectricity in layered In2Se3 nanoflakes. Soon afterward, a graphene/In2Se3 heterojunction was successfully utilized as a room-temperature ferroelectric diode, followed in 2019 by discovering a MoTe2 2D nanostructure that exhibited ferroelectric properties at room temperature.
Long-Range Ferromagnetic Order in Two Dimensions
Similar to the 2D ferroelectrics, the 2D ferromagnetic materials are attracting considerable interest from the scientific community. Ferromagnetic materials possess a permanent magnetic moment in the absence of an external magnetic field when the material's temperature is below the critical Tc.
Introducing a long-range magnetic order and strong enough magnetic anisotropy that can overcome the thermal fluctuation at temperatures relevant to practical applications proved extremely challenging in atomically-thin 2D nanomaterials.
Despite that, in 2017, researchers from the Massachusetts Institute of Technology and the University of Washington in the USA discovered the ferromagnetic properties of 2D monolayers of CrI3. At the same time, a research group from the University of California in Berkeley demonstrated 2D ferromagnetism in Cr2Ge2Te6 2D nanocrystals. Although both materials behaved as 2D ferromagnetics only at temperatures below 45 K, these results stimulated extensive theoretical and practical research efforts in the field.
2D Heterostructures for Memory Applications
Due to the non-volatile nature of their spontaneous polarization and magnetic anisotropy, the 2D ferromagnetic and ferroelectric materials were identified as a promising concept for the development of non-volatile memory devices. Since the thickness of 2D nanomaterials is only a few atomic layers, it is much easier (compared to conventional bulk semiconductors) to modulate their electrical and magnetic properties through external electric and magnetic fields. This, in turn, offers possibilities to greatly reduce power consumption or even achieve near-zero power consumption.
Overcoming the Constraints of the 2D Nanomaterials
In the last five years, several proof-of-concept field-effect transistors employing 2D ferromagnetic and ferroelectric materials have been tested as initial steps toward the practical applications of these materials in electronics.
At present, the typical Tc of 2D layered ferromagnets is far too low for any practical applications. Another important challenge is to create air-stable 2D ferromagnetic and ferroelectric materials suitable for long-term operation under ambient conditions. The ongoing efforts aim to resolve these problems and establish large-scale fabrication methods for high-quality and low-cost 2D nanomaterials that can be used in ultrathin, low-power, and flexible memory devices.
Continue reading: Repelling Radiation with Carbon Nanotubes.
References and Further Reading
Liu, Z., et al. (2021) Ferromagnetic and ferroelectric two-dimensional materials for memory application. Nano Res. 14, 1802–1813. Available at: https://doi.org/10.1007/s12274-020-2860-3
Wu, M. and Jena, P. (2018) The rise of two-dimensional van der Waals ferroelectrics. WIREs Comput Mol Sci. 8:e1365. Available at: https://doi.org/10.1002/wcms.1365
Geim, A., Grigorieva, I. (2013) Van der Waals heterostructures. Nature 499, 419–425. Available at: https://doi.org/10.1038/nature12385
Disclaimer: The views expressed here are those of the author expressed in their private capacity and do not necessarily represent the views of AZoM.com Limited T/A AZoNetwork the owner and operator of this website. This disclaimer forms part of the Terms and conditions of use of this website.