For the first time, the functional properties of phosphorene nanoribbons in optoelectronic devices have been demonstrated experimentally. Here, we speak to Dr. Macdonald and Prof. Howard about their involvement with this 'wonder material', and how it could advance the performance of perovskite solar cells.
Please could you introduce yourself and tell us what initially inspired your investigation into the ‘wonder material’ that is phosphorene nanoribbons?
Prof. Howard (H): I am a Professor of Materials Physics at University College London. The aim of our research is to create and study new materials that are scientifically interesting, have technological use, or both. The phosphorene nanoribbons (PNR) discovery was serendipitous, an undergraduate project aiming to apply a method the group had pioneered for exfoliating layered materials in liquids to the cool new layered material on the street, black phosphorus, aiming for 2D sheets.
We had some issues exfoliating black phosphorus, but we tried different approaches and noticed ribbons rather than sheets. It took us another three years to optimize our method and characterize the ribbons, which turned out to be extraordinary for many reasons.
Meanwhile, and despite the fact discrete ribbons did not exist yet, the theoretical community calculated that these structures would have an array of fantastic properties from the fundamentally exotic to transformatively useful for applications.
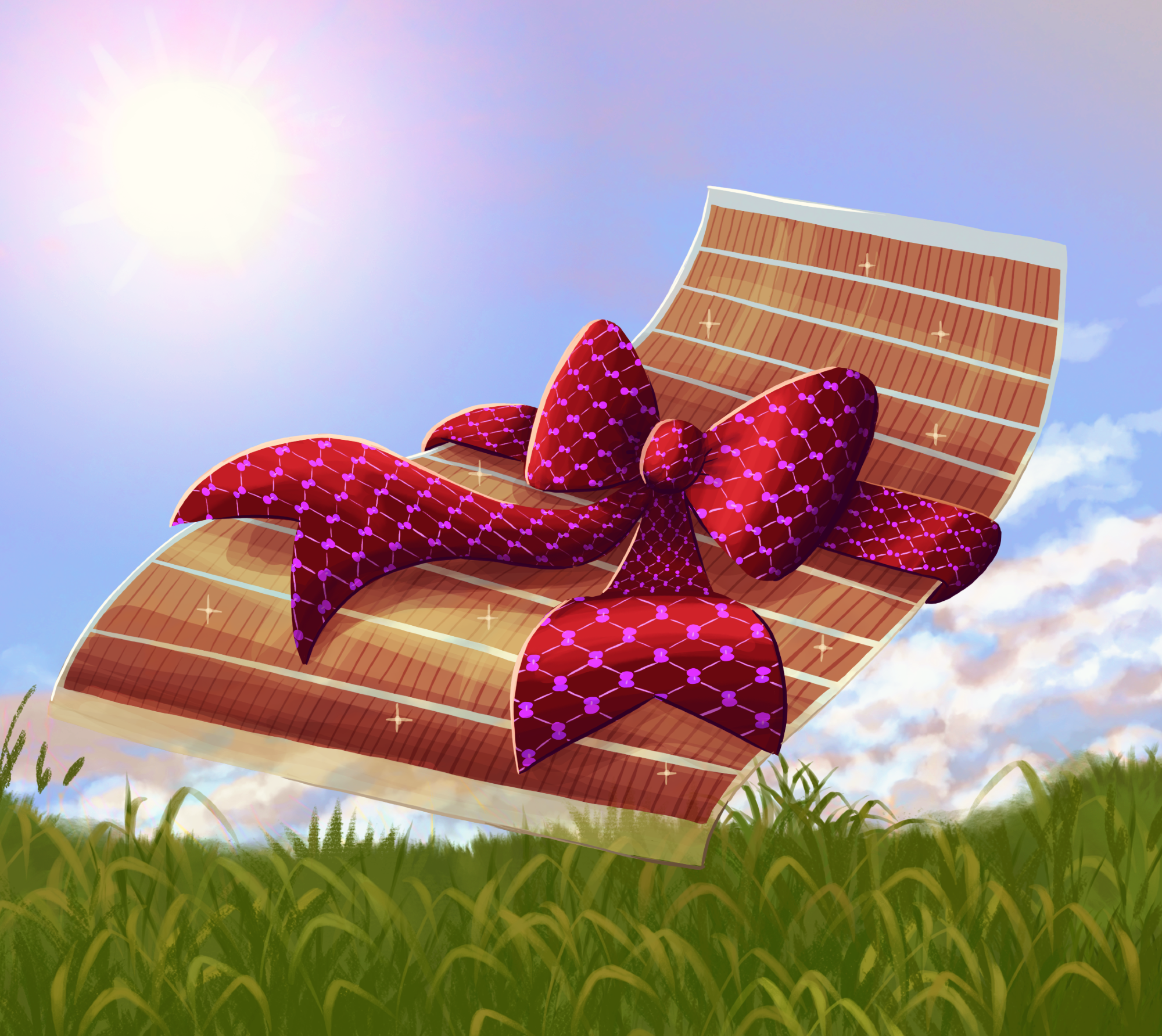
© Martina Pepiciello
Dr. Macdonald (M): I am an 1851 Research Fellow working on nanomaterials at Imperial College London. My research aims to bridge innovations in nanomaterial synthesis with application in solar energy conversion.
With Prof. Howard’s expertise in PNRs and my expertise in solar cells, we put our ideas together to see if we could establish some new and useful understandings for PNRs in optoelectronics. While we had obviously hoped for a positive result, we had no idea that the PNRs would be this effective, and it was great to definitively prove the origin of their performance enhancement in state-of-the-art solar cells.
How did you first become involved with perovskite solar cells?
M: Like most perovskite solar cell (PSC) researchers, it all started with dye-sensitized solar cells, where I still clearly remember being excited to reach efficiencies of just 8%! My interest in PSCs stems from two reasons.
Firstly, early discoveries showed that perovskites could independently conduct both electrons and holes. Secondly, their rapid development with solid-state hole transport materials where it seemed like efficiencies went from almost 10% to 15% overnight! I fabricated my first PSC in 2017, and almost five years later, I am still making devices today.
Only now, I get the pleasure of training graduate students in the fabulous art of device fabrication!
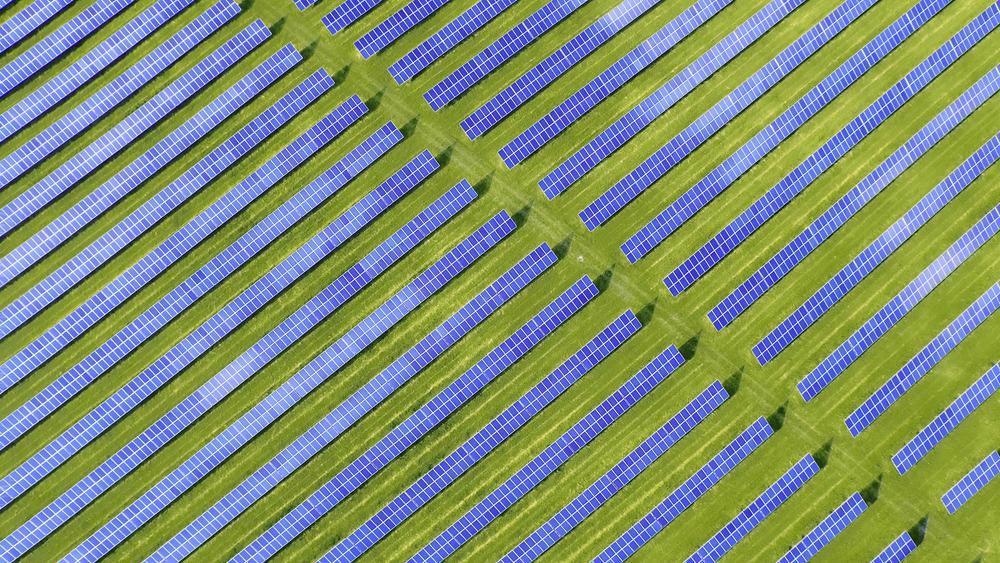
© Clever Stock/Shuttestock.com
In your own words, could you summarize the results of your research?
Over many years, phosphorene nanoribbons (PNRs) have been theoretically predicted to exhibit a range of exciting properties, yet until now, these properties have never been demonstrated in actual devices."
M: Our results show that the predicted functional electronic properties of PNRs indeed translate to improved performance in application, which highlights the genuine importance and utility of this newly discovered nanomaterial and sets the benchmark for PNR-based optoelectronic devices.
Could you briefly explain what phosphorene nanoribbons are, and why they are particularly suited to this field of research?
H: Phosphorene nanoribbons are ribbon-like strips of the 2D material phosphorene – itself a single layer of phosphorus atoms. Nanoribbons are an exciting class of materials with unusual characteristics arising from their 2D nature combined with the effect that their narrow widths have on their properties. Their edge terminations also result in unusual effects.
The morphology of the ribbons also lends themselves to be deposited into a high surface area mat relevant for many applications. The property we exploited here is the fact that charge carriers called holes can move extraordinarily fast in PNRs, making them a highly desirable additive layer that improves the performance of the solar cells we’re interested in.
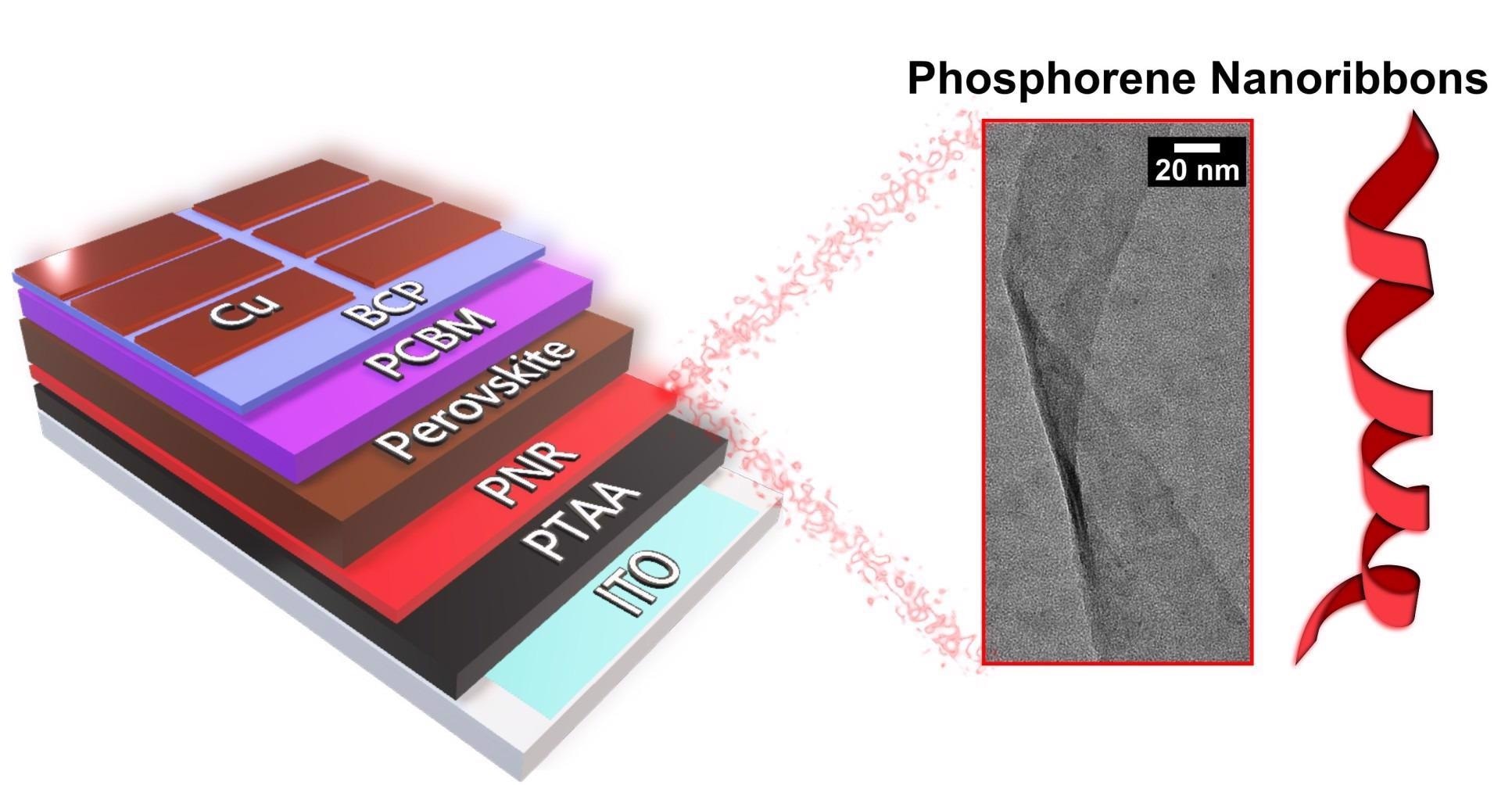
© T.J. Macdonald and T. Webb
This research experimentally demonstrated the exciting potential of PNRs for the first time. How did you approach achieving this?
M: Due to the air sensitivity of both perovskites and PNRs, we knew that we would have to spend a lot of time inside a glovebox to optimize the true potential of both perovskites and PNRs.
Aside from this, PNRs are typically dispersed in polar aprotic solvents (e.g. DMF), which are actually the same solvents used to make the perovskite precursor ink. This meant that we had to carefully develop our layers to ensure the solvents wouldn’t harm any other layers within the PSC.
This led us to use what we call the ‘inverted’ PSC architecture (or p-i-n cells) to incorporate the PNRs prior to the deposition of the active perovskite layer. The approach also provided us with the most appropriate energy alignment to transport charges between our contact layers in the device.
Did you come across any challenges during your research, and if so, how did you overcome them?
M: It may sound cliché, but the main challenge was overcoming the lockdowns with COVID-19, which caused countless delays between all the institutions and collaborators involved. For example, this project was affiliated with ten international institutions, so you can imagine the difficulties encountered while trying to navigate lockdowns with an experimental research team!
Perovskite solar cells are a hot topic in research and commercial communities worldwide. Have you noticed any particular trends developing across this field?
M: The most significant trend I have observed is the evolution of alternative hole transport materials. This includes using self-assembled monolayers, which can modulate the work function of transparent conducting oxides (e.g. indium tin oxide) for better control of the energy band alignment between the different materials and interfaces within a PSC.
The emergence of printable carbon-based contacts has also shown popularity over the past few years, where carbon inks can be printed on the perovskite or respective hole transport material as a cost-effective top contact. This reduces the reliance on costly thermal evaporation procedures, which typically incorporate metal top contacts such as gold or silver.
Interestingly, these developments all coexist in the area of ‘charge extraction’. As a result, our recent discovery on PNRs improving the hole extraction in PSCs is very timely and allows room for further development of this exciting field.
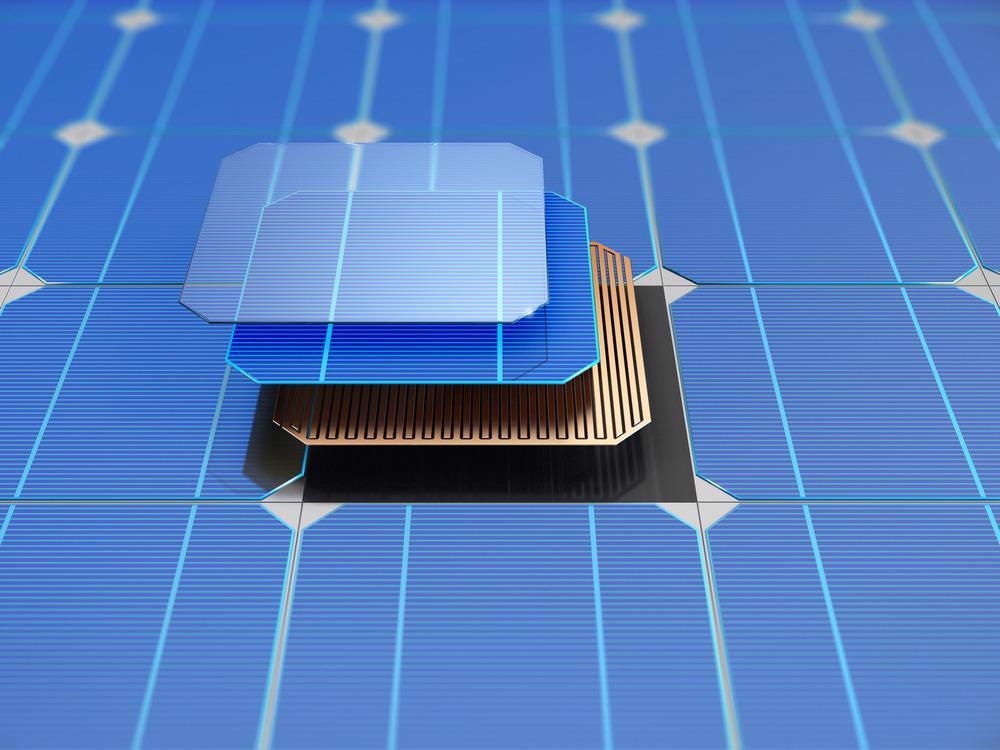
© Iaremenko Sergii/Shutterstock.com
Why is innovation in the field of solar cell research so important, and how might it help to increase the implementation of solar energy devices?
M: Innovation is important because it drives our curiosity to ask the question, ‘what else is out there?’, and thus learn about the potential benefits of unexplored materials (such as PNRs).
In particular, while this innovative study resulted in efficiencies on par with traditional silicon solar cells, the most important part of the study was identifying how PNRs could actually benefit a solar cell. Doing so enables future research into further developing this technology which, unlike traditional silicon cells, can provide fully solution-processable routes and hence is suitable for roll-to-roll and printable processing.
Leading innovations in solar cell research will help us discover more transformative advantages of nanomaterials, which will drive the development of new technologies to further benefit society in minimizing the use of fossil fuels."
According to your research, increased performance is due to the fact that PNRs improve ‘hole mobility’ in cells. Could you explain ‘hole mobility’?
M: ‘Holes’ are the opposite partner of electrons in electrical transport, so improving their mobility (a measure of the speed at which they move through the material) helps electrical current move more efficiently between layers of the device.
How might the PNRs be modified in the future to further their capabilities?
M: Modifying the surface chemistry of the nanoribbons could further improve the unique electronic properties of our proposed system. For instance, modifying the PNR surface chemistry so that the materials can form chemical bonds with the other constituents in the solar cell could result in even further improvements in performance.
What does the future of this novel nanomaterial look like to you? Are there any other sectors that would benefit from its use?
H: We’re very excited by the future for PNRs, they have a very wide number of exciting and useful properties predicted, and we’re starting to see some of these come through strong in our research.
Other groups are coming up with new methods for making PNRs, which is superb for the field, but our method is the one that produces ribbons with the narrowest widths, which are essential for the emergence of a number of important properties. We have some exciting work in the pipeline, which we will publish next year.
What are the next steps for this field of research?
M: The next step will be isolating the PNRs as a sole hole-transport material for state-of-the-art devices. It’s also important to highlight that the exciting properties of PNRs are not be limited to solar cells, and we are also working on getting these materials into both light-emitting-diodes and batteries.
Thanks to perovskites you will throw away your chargers | Olga Malinkiewicz | TEDxWroclaw
Thanks to perovskites you will throw away your chargers | Olga Malinkiewicz | TEDxWroclaw © TEDx Talks/YouTube.com
What aspect of this research do you find particularly exciting?
H: The ribbons have been such a pleasure to study. Firstly, they are beautiful and unusual materials in their own right. Furthermore, these single crystals with aspect ratios similar to the cables that run the length of the Golden Gate Bridge are incredibly flexible. But what’s really exciting is just how many areas the ribbons show exciting potential from theoretical prediction.
This allows us to collaborate with many experts, and my collaboration with Dr. Macdonald is one great example. In this collaboration, we not only measured exciting properties but also demonstrated that they translate to excellent performance in devices.
Where can our readers go to follow the progression of this research?
H: Please keep updating #PhosphoreneNanoribbons and #PNRs across social channels, and we will also try and promote and explain our work beyond the academic journals.
M: I also urge readers to keep a close eye on the field of PNRs in optoelectronics since this study was only the tip of the iceberg.
While we’ve taken the first step to getting these materials into solar cells, there are many more exciting steps to take which will further drive the usefulness of this material in devices. Future work integrating PNRs into different types of electronic devices will provide exciting insights into new innovations in nanomaterial research.
Check out some of our research here, with our most recent research highlighted at the top:
About Dr. Thomas Macdonald
Thomas J. Macdonald is an 1851 Research Fellow in the Department of Chemistry at Imperial College London. He was previously a Visiting Research Fellow at University College London, University of Queensland, and Flinders University. Before this, he was a Ramsay Memorial Fellow at University College London working on nanomaterials for solar energy conversion. He has a strong interest in renewable energy and extensive experience in the synthesis of functional nanomaterials and the fabrication of third-generation photovoltaics.
About Prof. Chris Howard
Chris Howard is a Professor of Materials Physics at University College London where he had previously obtained his Ph.D. The main aim of his lab is to create new materials that have desirable functional properties or that exhibit interesting emergent phenomena and he studies these materials with a wide range of experimental techniques. Outputs range from nano-textured electrodes for battery and fuel cells to the discovery of exotic electronic groundstates.
Disclaimer: The views expressed here are those of the interviewee and do not necessarily represent the views of AZoM.com Limited (T/A) AZoNetwork, the owner and operator of this website. This disclaimer forms part of the Terms and Conditions of use of this website.