Solar cells are pinned as one of the key sources of renewable energy; however, commercial options remain reliant upon silicon solar cells. In this interview, we speak with Professor Jacek Jasieniak about a nanoparticle ink used to produce low-cost printable perovskite solar cells, helping to catalyze the technology transition towards perovskite-based devices.
Could you introduce yourself to our readers and tell us about your current position and research activities?
My name is Jack Jasieniak and I am a material science and engineering professor at Monash University. I am also the Pro Vice-Chancellor of research infrastructure at Monash. My research is about developing next-generation materials for new energy technologies as well as advanced sensing applications.
Our most recent activity is a collaboration between Monash University and the Commonwealth Scientific and Industrial Research Organisation (CSIRO), who is a key partner focused on achieving large-scale printed perovskite devices.
Could you summarize the results of your recent research?
When you look at a solar cell in general, it is a multilayered device; a perovskite device generally has five layers. It is important to understand the materials in each of those layers and how each of those layers is then interfacing. Recently, we have focused on a layer called the electron transport layer, which allows electrons to be selectively transported out of a solar cell material.
For perovskite devices, you have a metal halide perovskite as the absorbing layer, which is sandwiched between two charge selective layers, one for electrons (negative charges) and the other for holes (positive charges), and capped with appropriate electrodes. Once you absorb light, it generates electrons and holes within the perovskite which then have to be effectively conducted through the appropriate charge transport layer to reach the electrodes. So, the electron transporting layer plays a significant role in defining half of the operation of that device.
Developing such a layer that is printable, scalable, and stable is critical, and something we have been able to achieve.
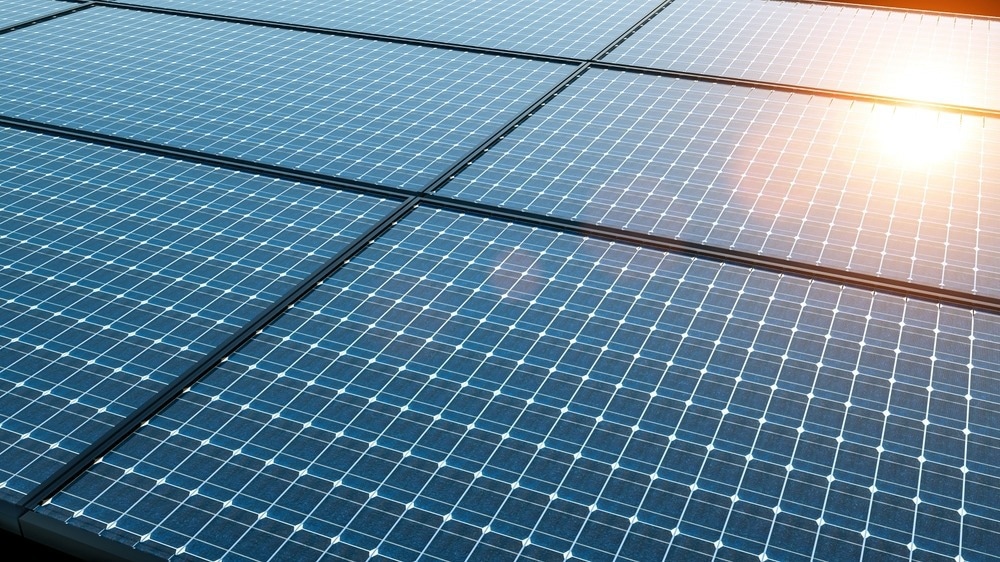
Image Credit: Audio und werbung/Shutterstock.com
What are nanoparticle inks?
Nanoparticle inks are formulations whereby you have nanocrystals dispersed in a particular liquid along with appropriate stabilizers and additives to allow for it to be printed and deliver the right kind of structure in its printed form.
Now, we have been looking at taking a ‘one pot approach’ to developing easy-to-synthesize inks. In this particular case, we have been focussed on developing tin oxide inks using tin-based salts in an alcohol mixture composed of benzyl alcohol and a little bit of ethanol to provide some additional polarity.
Then we applied microwaves to this salt solution to induce heat, which drove a chemical reaction and the formation of tin oxide.
The beauty of this reaction is that the microwaves induce this transformation. The benzyl alcohol absorbs onto the surface of the nanocrystals and provides a neat passivation layer, which prevents the nanoparticles from effectively agglomerating and allows for their dispersion. In doing that, you effectively have a rapid and very high conversion rate that is also very facile.
On top of that, we have analyzed how you can use this ink directly in your printing process. So not only have you made your particles, but you have made a dispersion that is very, very stable. You can take it, dilute it up with appropriate solvents, and then use it directly in your printer. In our case, we used slot-dye printing to demonstrate the concept.
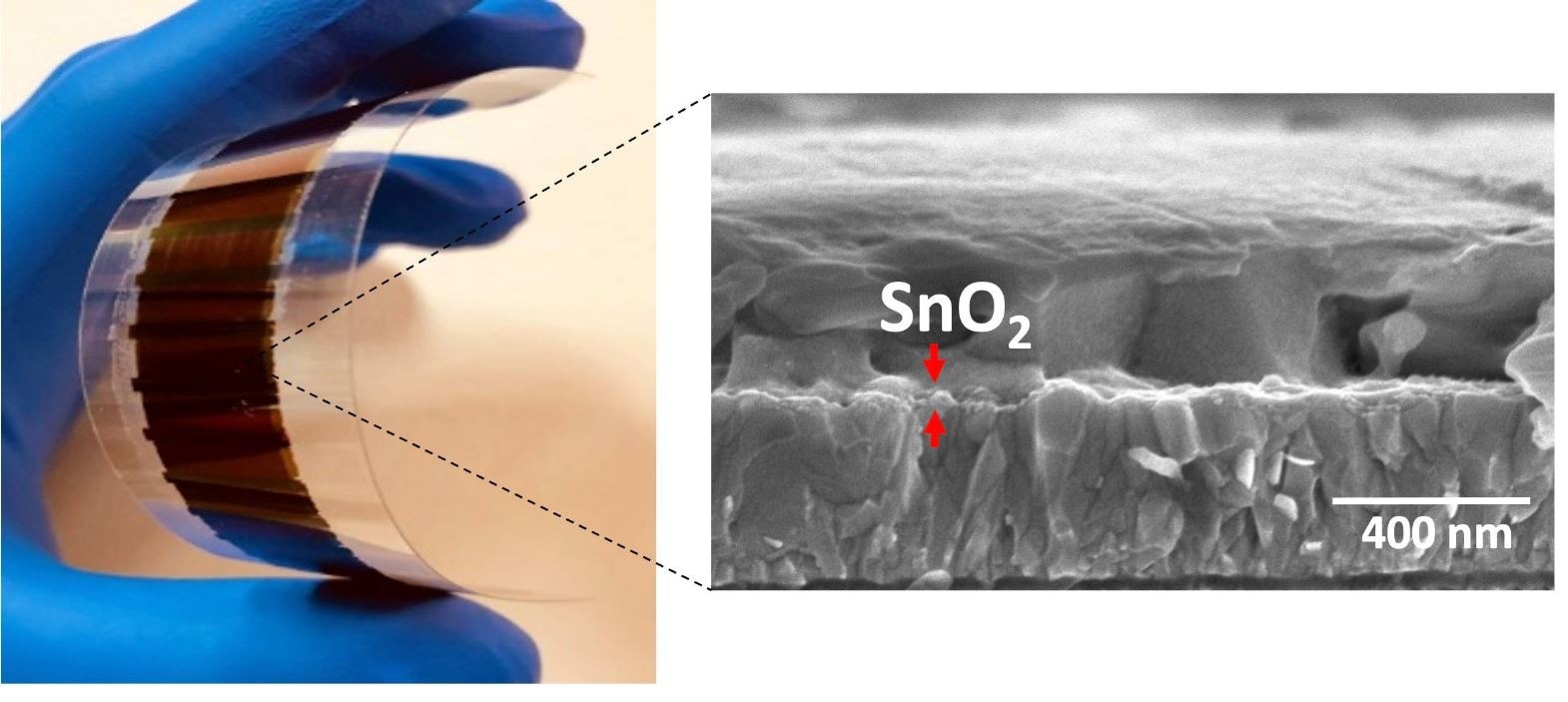
Printed perovskite devices (left) and the cross-section electron micrograph showing the individual layers, (right), including the thin tin oxide electron transport layer. Image Credit: Jacek Jasieniak
The slot-dye method, also known as roll-to-roll is typically associated with newspaper printing; why was this approach used to fabricate solar cells?
Slot-dye is a straightforward approach in that it allows the ink to be effectively flown through a nozzle that is placed above a moving printing surface. By controlling the movement rate of the substrate, the nozzle height, as well as the rate of the liquid addition, you can control layer thickness within the device to a high degree.
Doing so produces a scalable approach to obtaining simple structures suitable for thin film solar cells integrated as modules.
This is because printed modules are effectively multi-layered structures that are just a little bit offset on some of the layers. Slot-dye allows you to print such simple linear features and offsetting is relatively straightforward.
Did the team face any challenges or limitations in using this approach?
There are certainly some challenges in using the slot-dye method. For example, you need to get the ink formulations well developed so that it allows the flow and drying conditions to be right and interface to the subsequent layers.
Despite this, slot dye is a very versatile approach and is seen as a really progressive approach to developing thin film solar cells using printing approaches.
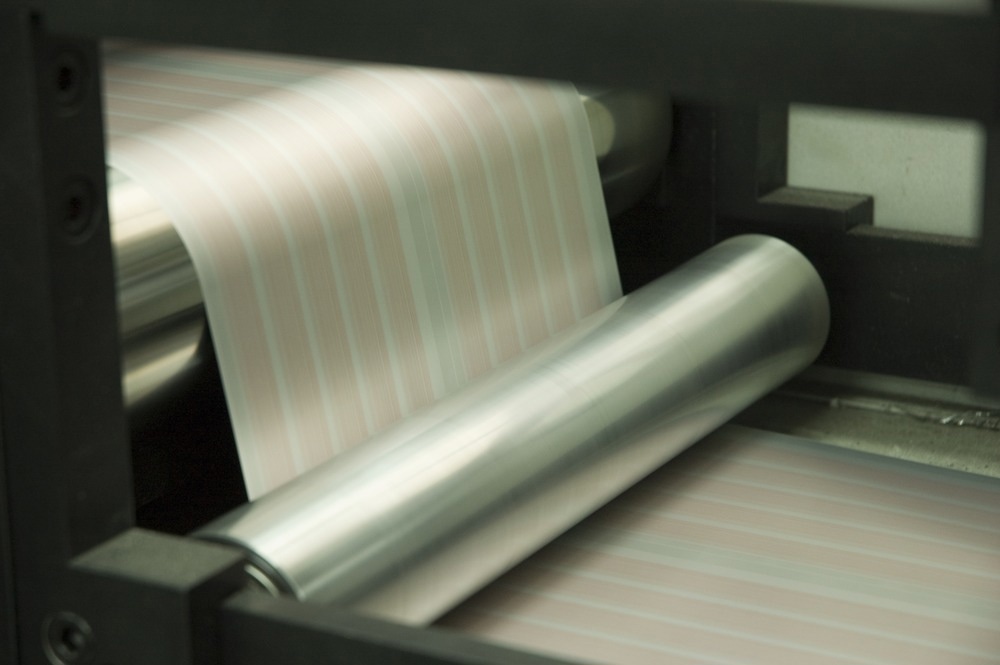
Image Credit: DJ Srki/Shutterstock.com
Metal halides are cited as very promising materials for solar cells; what properties of tin oxide, in particular, made it especially suitable for this research? Would you consider any other materials in future research?
Tin oxide is a suitable charge transport material because it has a high lattice energy, making it very stable as a chemical compound. It also crystallizes at a modest temperature making it feasible for these types of reactions.
Because of its high electron mobility, tin oxide can charge transport very effectively and acts as selective electron transport material very well. Its energy levels are appropriate for use in metal halide perovskite solar cells, particularly those with a band gap in the near-infrared.
There are alternative materials that people routinely use for electron transport layers. TiO2 (titania) is one example, but it requires a much higher temperature to crystallize. This is a challenge to overcome, so if one could do it at lower temperature, that would be beneficial for printing. It is hard to say if titania would ultimately be a better or worse material for printed perovskite solar cells, but it would certainly be an interesting comparison.
What do you think are the main barriers that limit the translation of research into high-performance perovskite solar cells to actual industrial practice and mass manufacture?
Most of the research into perovskites is done on devices that are not compatible with mass manufacturing.
As a result, a lot of innovation is focused around developing high efficiency, sometimes high stability devices using techniques that, quite frankly, do not have relevance to scale devices. A practical example is the deposition of inks deposited using a conventional small-scale method, like spin coating, versus something that is completely scalable like slot-dye coating. These two approaches with the same ink will exhibit different flow characteristics, drying characteristics, and final structure properties.
I think there needs to be a transition in this entire field that moves away from a focus on purely high efficiency to a focus on high efficiency, using relevant techniques that allow realistic scalability.
However, while this transition is important, it is not easy for the research community to make because these techniques are, quite frankly, much more challenging to deposit and master.
As such, this movement really does rely on key partnerships with labs that have access to and expertise in this equipment, as well as an understanding on how quickly we can translate small-scale device development to scalable techniques.
How did your partnership with CSIRO begin, and what benefits has it brought to this research?
Before joining Monash in 2015, I was a group leader at CSIRO, the Commonwealth Scientific and Industrial Research Organization.
It is a research government lab here in Australia and is focused largely on translational applied research to tackle industry problems and take devices to commercial stages. I already had really strong links to CSIRO, particularly in the team that I used to work in, which was around printed electronics.
In this particular area, we joined forces to look at the advances around printed perovskites through facile processing, which means no special environments.
Partnerships with organizations like CSIRO are critical because they bring that industry perspective. In this case, they brought their prototype, slot-die systems with roll-to-roll processing units with integrated drying, heating, and monitoring provisions. Such a design enables the bridge between small and large scale to be crossed, which I think is a really big step.
What are the next steps for this research?
One of the areas that we have an active program in is solar window technologies. Solar windows can leverage from similar types of structures, perovskites, and they absolutely can leverage from the printing approaches that we have demonstrated this ink on.
The next body of work will look at understanding how we can develop printed structures that can act as solar window technologies. As the first point of call, these may be as a laminate or a more integrated feature of a double glazed configuration. It does not have to be flexible, but it has to have that functionality of high efficiency while enabling sufficient visible light to come through with long term stability.
There is a huge market in this space that is exponentially increasing. By 2025, reports suggest that this market will grow to be of the order of five, $6 billion, USD. This is a niche market compared to global photovoltaics like silicon, but it is a market that will continue to grow.
About Professor Jacek Jasieniak
Jacek Jasieniak completed a Bachelor of Science (1st Class Honours) from Flinders University (2003, University Medal) and Ph.D. from the University of Melbourne (2008, Chancellor’s Prize) under the supervision of Prof. Paul Mulvaney. He then undertook postdoctoral work as an OCE fellow at the Commonwealth Scientific and Industrial Research Organisation (CSIRO) with Dr. Scott Watkins and Dr. Ezio Rizzardo (2008-11), and was a Fulbright Fellow with Nobel Laureate, Prof. Alan Heeger, at the University of California Santa Barbara (2011-12). In 2012 he returned to CSIRO, progressing to a Senior Research Scientist and then Group Leader.
In 2015 he moved to Monash University as an Associate Professor, where he was also appointed Director of the cross-disciplinary Monash Energy Institute. In 2020 he was promoted to Full Professor and also became the Associate Dean of Research for the Faculty of Engineering. In December 2021, Jacek was promoted into the role of Pro Vice-Chancellor (Research Infrastructure) at Monash University.
His research interests include the development of nanomaterials and their use in various next-generation energy technologies to enable a net-zero carbon emission future. Towards this goal, he has worked on various functional material classes to develop microlasers, light emitting devices, printable solar cells, batteries, X-ray detectors and a variety of chemical sensors.
His contributions have been broadly showcased across newspapers, radio, television and online blogs to reach audiences of millions of people across topics ranging from energy poverty, to smart phone batteries, developing solar windows and creating net-zero cities through large scale solar cell deployment.
Disclaimer: The views expressed here are those of the interviewee and do not necessarily represent the views of AZoM.com Limited (T/A) AZoNetwork, the owner and operator of this website. This disclaimer forms part of the Terms and Conditions of use of this website.