In this interview, we speak with CityU's Dr.Zhiyuan Zeng about a new approach to in situ liquid cell transmission electron microscopy that holds great promise for the real-time viewing of complex electrochemical reactions at the nanoscale.
Please could you introduce yourself and your research activities?
I am an Assistant Professor at the Department of Materials Science and Engineering, City University of Hong Kong (CityU). I obtained my Ph.D. from Nanyang Technological University, Singapore in 2013.
After postdoctoral training at Lawrence Berkeley National Laboratory (2013-2017), followed by Senior Process Engineer in Applied Materials Inc. (2017-2019, Silicon Valley), I joined CityU in 2019.
My research interests include in-situ liquid phase TEM for real-time tracking of dynamic electrochemical processes, as well as electrochemical lithium intercalation and exfoliation method for 2D transition metal dichalcogenides (TMDs) preparation. I am also interested in energy and environmental applications such as batteries, membranes, and heavy metal removal, etc.
What is the motivation behind developing improved liquid cells for electrochemical TEM studies?
Real-time visualization of complex electrochemical reactions at the nanoscale is essential to understanding these reactions fundamentally. In situ electrochemical liquid phase transmission electron microscopy (TEM) offers an opportunity for directly visualizing and analysing these reactions.
However, current electrochemical TEM methods are based on commercial electrochemical liquid cells, which are limited by their low imaging resolution. Therefore, this has prompted us to develop an advanced liquid cell with higher resolution than commercial ones.
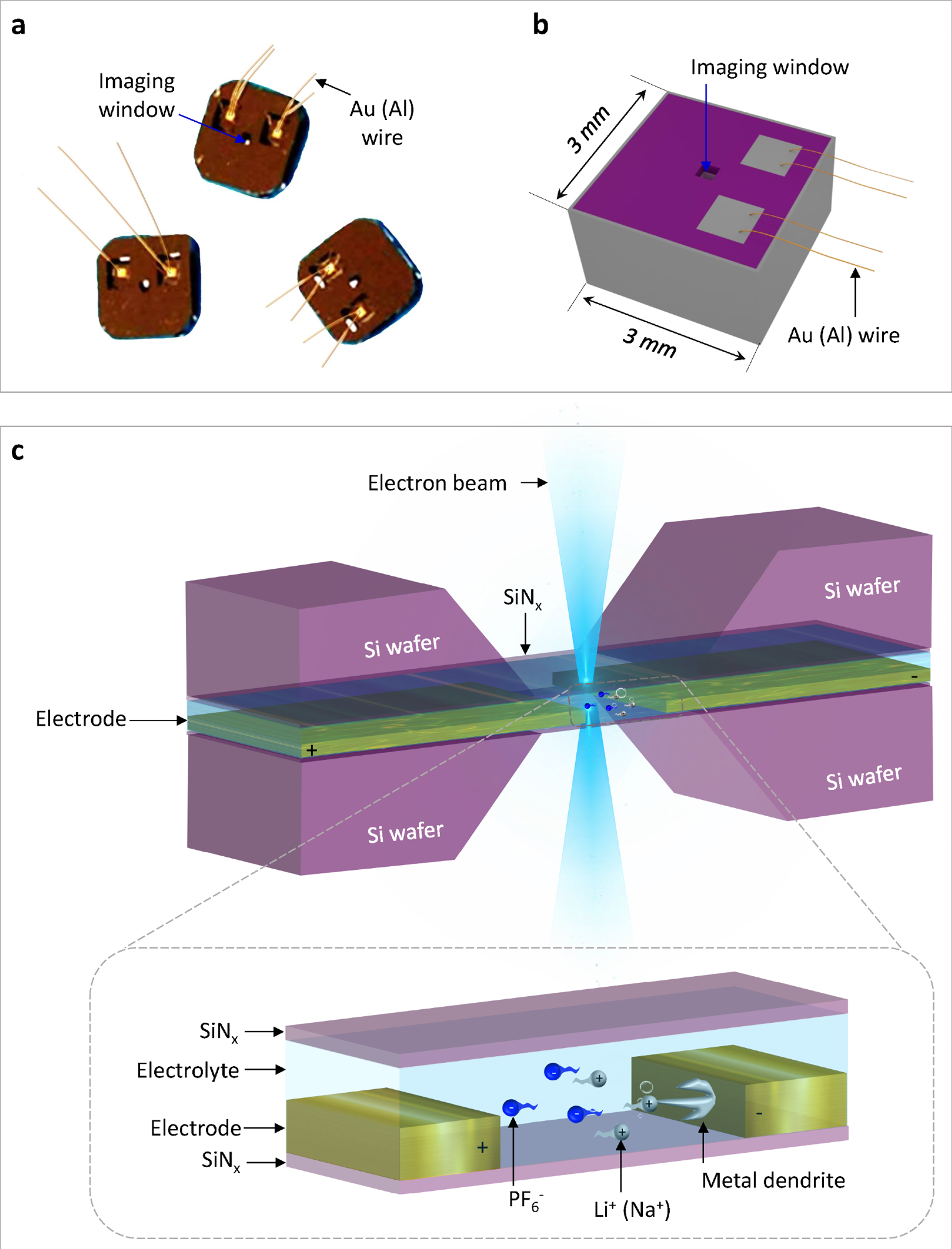
Schematic illustration of the electrochemical liquid cell. (© Yang, R. et al. https://www.nature.com/articles/s41596-022-00762-y)
Why are in situ observations so significant for researching and designing energy devices?
Traditional ex situ TEM observations of electrochemical reactions are limited by the need to remove samples from their native environments and by the time delay between the reaction and the observations. This spatial asynchrony and time lag hinder the research and understanding of the authenticity of the electrochemical reaction in energy devices.
Therefore, obtaining real-time and real-environment information about the electrochemical reactions and processes inside energy devices without disassembling them is vital. Gaining this information significantly contributes to the design of more efficient energy devices.
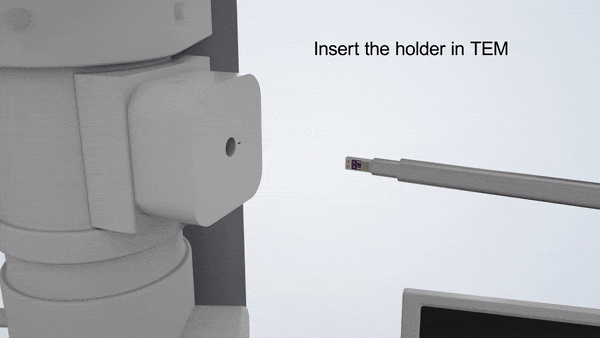
In-Situ TEM Operation. In situ TEM observation of electrochemical processes and post-in situ characterizations. (© Yang, R. et al. https://www.nature.com/articles/s41596-022-00762-y)
Could you discuss the new protocol that was recently published in nature protocols?
In this protocol, we described the detailed procedures of our established technique, that is, photolithography-assembly technology, for fabricating electrochemical liquid cells for in-situ TEM observation. In addition, a detailed operation procedure for the in-situ TEM observation of electrochemical reactions using the nanofabricated electrochemical liquid cell was also presented.
The captured dynamic electrochemical reactions using the fabricated electrochemical liquid cells were also demonstrated for many mechanism studies, such as lithium dendritic growth and solid electrolyte interface (SEI) formation, electrochemical alloying and de-alloying of gold anodes, electrochemical lithiation and delithiation of molybdenum disulphide nanosheets. This technique opens a venue by which to look inside the electrochemistry at an unprecedented level, thus improving electrode design for reducing short-circuit failure and improving the performance of lithium-ion batteries.
How does this method differ from existing workflow using commercial liquid cells? What benefits does this bring?
If comparing our developed technology and commercial technology for the fabrication of electrochemical liquid cells, the differences are reflected in the growth process of silicon nitride and the spacer. The electrochemical liquid cell designed by our nanofabrication method possesses thinner SiNx imaging windows (35 nm) than commercial ones (50 nm).
It also possesses a thinner liquid layer (150 nm) created by a thin indium spacer in contrast to that of commercial products (1,000 nm) created by an O-ring. The thinner SiNx imaging windows and thinner liquid layer ensure that our fabricated liquid cell can capture electrochemical reactions with better TEM spatial resolution than the commercial ones.
Are there any electrochemical systems where this technology that would be especially applicable?
In our previous work, several electrochemical systems were studied by in-situ TEM observation using our fabricated liquid cell, such as system I (2 gold electrodes, commercial LiPF6/EC/DEC electrolyte), system II (titanium electrode/MoS2 electrode, commercial LiPF6/EC/DEC electrolyte), and system III (2 titanium electrodes, NaPF6/PC electrolyte).
All two electrode electrochemical systems, such as lithium-ion batteries and sodium-ion batteries, are suitable for this technology.
What properties of silicon nitride make it especially suitable for use in the imaging window?
Silicon nitride is an ideal window material due to its high hardness, good electron transmittance, thin layer thickness, and strong corrosion resistance.
High hardness, for example, helps to avoid window breakage during use, even under very thin thickness, while good electron transmittance can ensure the high imaging resolution of the TEM.
Strong corrosion resistance can prevent it from being corroded by the electrolyte during the electrochemical reactions.
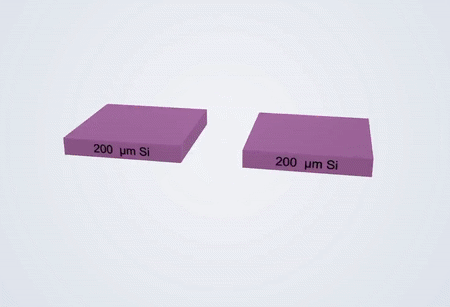
Fabrication process of the electrochemical liquid cell. (© Yang, R. et al. https://www.nature.com/articles/s41596-022-00762-y)
Could any other materials be used as the window instead of silicon nitride?
Graphene is an alternative window material for liquid cells beyond silicon nitride. The thin nature of the graphene window allows a high resolution for the liquid cell TEM. However, integrating electrodes into graphene liquid cells is challenging. Therefore, graphene windows are currently difficult for the fabrication of electrochemical liquid cells.
During fabrication, did the team encounter any problems, such as cell leakages? How were these resolved?
We have indeed encountered some problems in the manufacturing process. These problems and their solutions have been summarized in the Table 1 of our recent protocol published in Nature Protocols (Nat. Protoc. 2023, 18, 555-578). Among them, cell leakage is a common problem, which is normally caused by the deficient epoxy coating on the side of the liquid cell.
The solution to this problem is to carefully check the coating on the sides of the liquid cell by using an optical microscope to ensure that there are no holes on every side of the liquid cell.
How does the resolution of these cells compare to that of solid-state cells?
In our opinion, the resolution of our liquid cell is comparable with solid-state cells. However, liquid cell battery reactions are more representative of the real lithium ion and sodium ion battery reactions. Since the active electrode materials are immersed in a liquid electrolyte, full contact between the electrolyte and every side of the particles is ensured.
However, in in-situ solid-state cells, the solid electrolyte is not representative of the real solid electrolyte. Normally, the solid electrolyte used in in-situ solid-state cells are lithium oxide and ionic liquid, but in real solid-state cells, the solid electrolyte uses other materials rather than lithium oxide and ionic liquid.
Are there any challenges that remain regarding liquid cells that prevent a higher resolution from being achieved?
Indeed, reducing the thickness of silicon nitride can increase its electron transmittance, thus increasing the TEM imaging resolution. However, with the decrease in silicon nitride thickness, its mechanical strength will also decrease. Therefore, the thickness of silicon nitride should be greater than 35 nm to prevent breakage. This limits the further improvement of the imaging resolution by using this liquid cell.
Finding window materials with higher mechanical strength and better electron transmittance than silicon nitride is an effective way to improve the imaging resolution of the liquid cell.
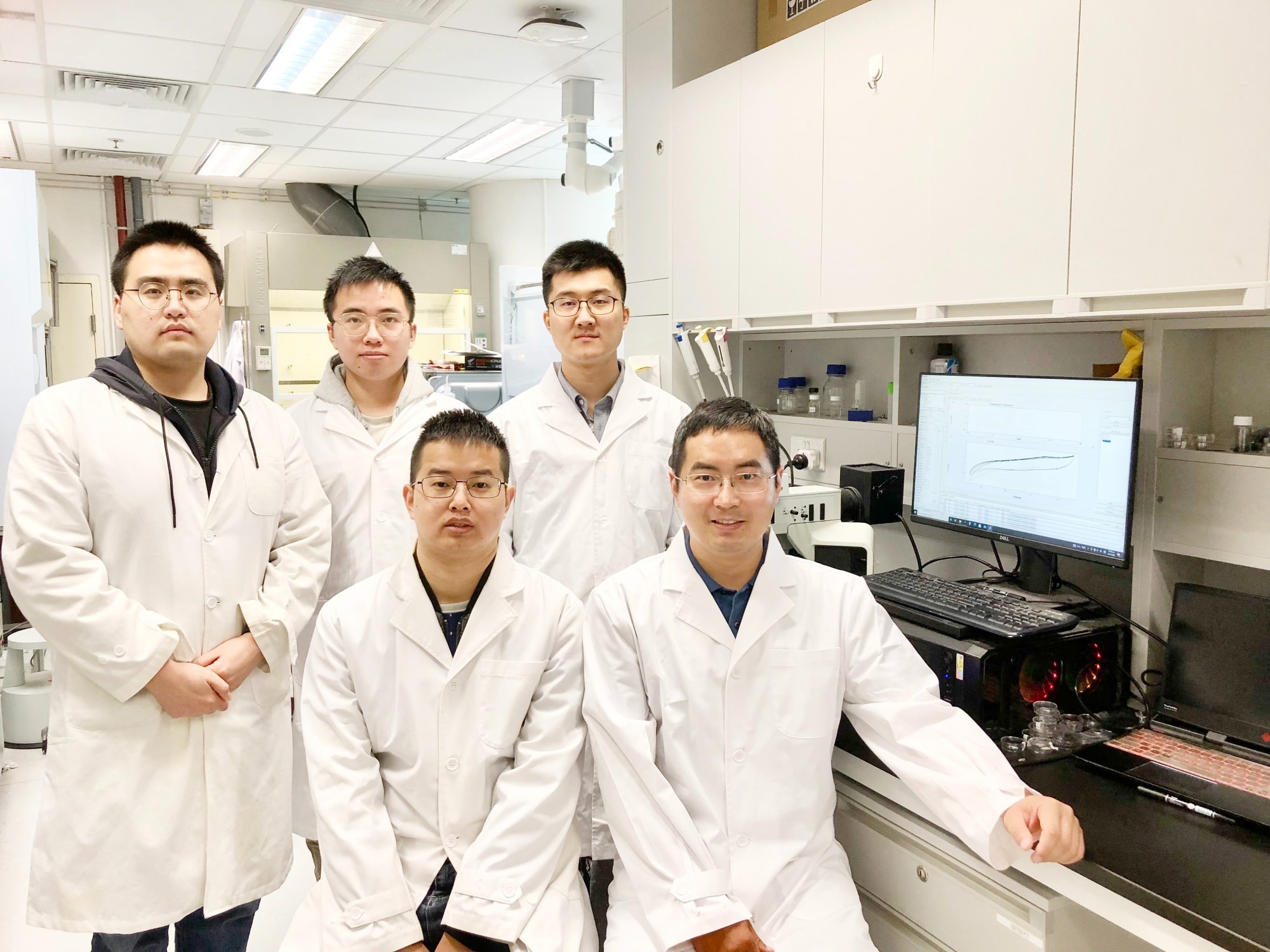
Dr. Zeng Zhiyuan (front row, right) and his research group from the Department of Materials Science and Engineering at City University of Hong Kong. (© City University of Hong Kong)
What are the next steps for this research?
In the next step, we are interested in extending this research in two directions. The first direction is on-demand fabrication of a series of structurally similar but functionally diverse electrochemical TEM liquid cells for the in-situ study of different electrochemical reactions. To achieve this goal, we need to adjust the types of deposited electrodes and the encapsulated liquid electrolytes as needed.
The second exciting direction is to extend this technology to manufacture electrochemical liquid cells for other in-situ characterization technologies beyond in-situ TEM, such as in-situ X-Ray absorption spectroscopy, in-situ X-Ray photoelectron spectroscopy, and in-situ X-Ray diffraction.
About Dr. Zhiyuan Zeng
Dr. Zhiyuan Zeng received his BSc, MPhil and Ph.D. degrees all in Material Science & Engineering from Central South University, Zhejiang University and Nanyang Technological University in 2006, 2008 and 2013, respectively. He continued his postdoc training at Lawrence Berkeley National Laboratory (2013-2017). Then he jumped into industry in Silicon Valley working for Applied Materials Inc. as a Senior Process Engineer (2017-2019).
Dr. Zeng joined CityU-MSE in 2019. His research interests are using battery intercalation strategy, in-situ liquid phase TEM to investigate TMDs and TMOs, which can be used for energy and environmental applications. He has published 111 SCI papers (72 IF>10) with a total citation of 18360 + times and H-index 53 (google scholar); 21 Papers were listed as ESI Highly Cited Papers, which include Nat. Mater., Nat. Protoc., Nat. Synth., Nat. Commun., Matter, Adv. Mater., Angew. Chem. Int. Ed., Nano Lett., etc.
He has been listed as the Highly Cited Researcher (Top 1%, Clarivate Analytics) in 2022, 2020 and 2018. He has been listed as the World's Top 2% Scientists in Nanosci. & Nanotechnol. in years 2022, 2021 and 2020 (by Stanford University). Dr. Zeng's other awards include the Rising Star by Advanced Materials (2022) and Small (2022), the Emerging Investigator by Chemical Communications (2021) and Journal of Materials Chemistry A (2020), the Early Career Scheme award by Research Grants Council of Hong Kong (2019).
Disclaimer: The views expressed here are those of the interviewee and do not necessarily represent the views of AZoM.com Limited (T/A) AZoNetwork, the owner and operator of this website. This disclaimer forms part of the Terms and Conditions of use of this website.
In this interview, we speak with CityU's Dr.Zhiyuan Zeng about a new approach to in situ liquid cell transmission electron microscopy that holds great promise for the real-time viewing of complex electrochemical reactions at the nanoscale.
Please could you introduce yourself and your research activities?
I am an Assistant Professor at the Department of Materials Science and Engineering, City University of Hong Kong (CityU). I obtained my Ph.D. from Nanyang Technological University, Singapore in 2013.
After postdoctoral training at Lawrence Berkeley National Laboratory (2013-2017), followed by Senior Process Engineer in Applied Materials Inc. (2017-2019, Silicon Valley), I joined CityU in 2019.
My research interests include in-situ liquid phase TEM for real-time tracking of dynamic electrochemical processes, as well as electrochemical lithium intercalation and exfoliation method for 2D transition metal dichalcogenides (TMDs) preparation. I am also interested in energy and environmental applications such as batteries, membranes, and heavy metal removal, etc.
What is the motivation behind developing improved liquid cells for electrochemical TEM studies?
Real-time visualization of complex electrochemical reactions at the nanoscale is essential to understanding these reactions fundamentally. In situ electrochemical liquid phase transmission electron microscopy (TEM) offers an opportunity for directly visualizing and analysing these reactions.
However, current electrochemical TEM methods are based on commercial electrochemical liquid cells, which are limited by their low imaging resolution. Therefore, this has prompted us to develop an advanced liquid cell with higher resolution than commercial ones.
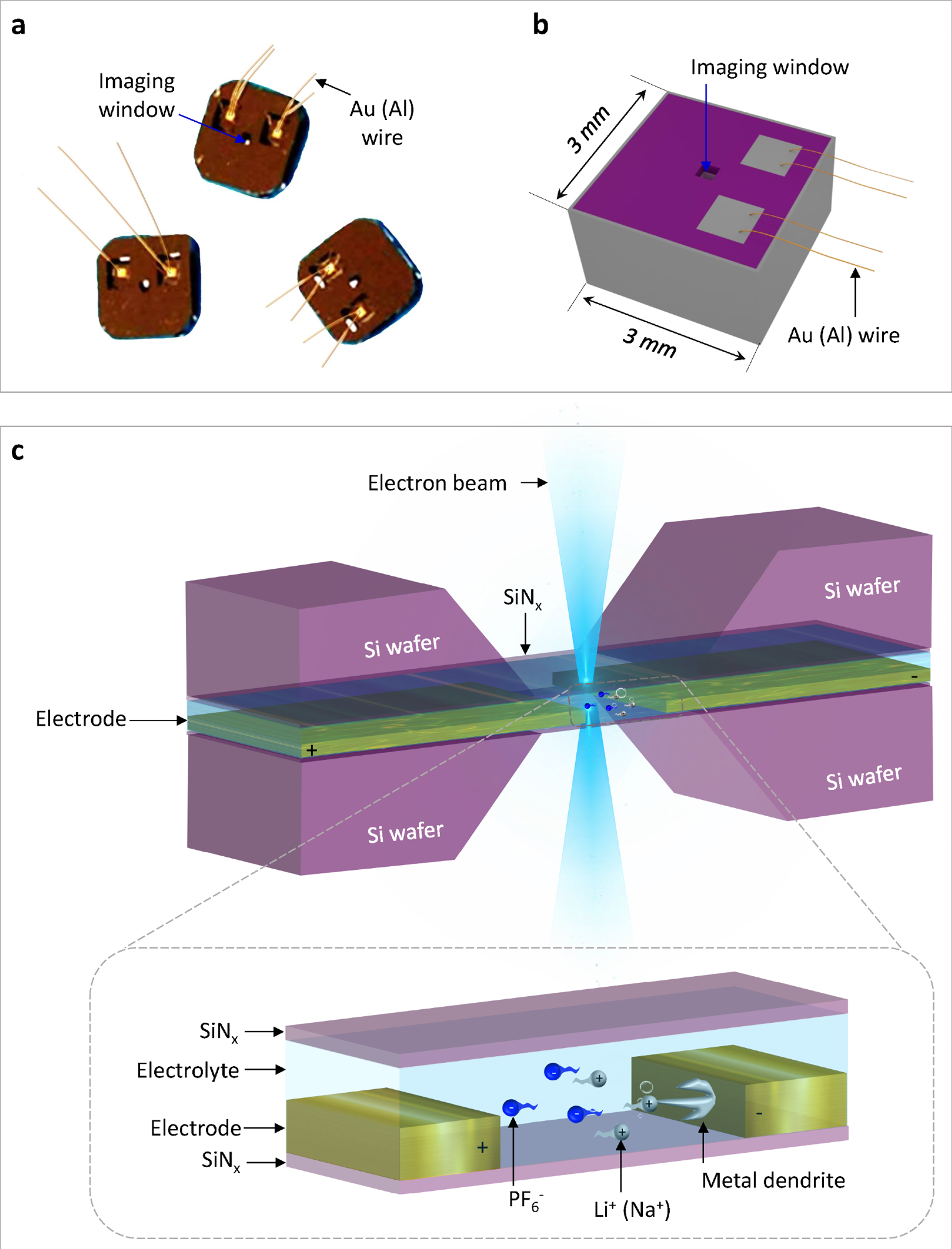
Schematic illustration of the electrochemical liquid cell. (© Yang, R. et al. https://www.nature.com/articles/s41596-022-00762-y)
Why are in situ observations so significant for researching and designing energy devices?
Traditional ex situ TEM observations of electrochemical reactions are limited by the need to remove samples from their native environments and by the time delay between the reaction and the observations. This spatial asynchrony and time lag hinder the research and understanding of the authenticity of the electrochemical reaction in energy devices.
Therefore, obtaining real-time and real-environment information about the electrochemical reactions and processes inside energy devices without disassembling them is vital. Gaining this information significantly contributes to the design of more efficient energy devices.
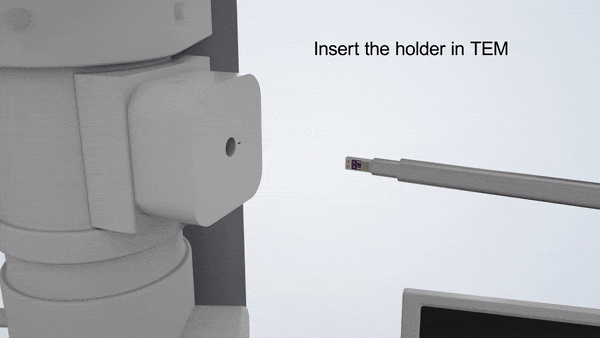
In-Situ TEM Operation. In situ TEM observation of electrochemical processes and post-in situ characterizations. (© Yang, R. et al. https://www.nature.com/articles/s41596-022-00762-y)
Could you discuss the new protocol that was recently published in nature protocols?
In this protocol, we described the detailed procedures of our established technique, that is, photolithography-assembly technology, for fabricating electrochemical liquid cells for in-situ TEM observation. In addition, a detailed operation procedure for the in-situ TEM observation of electrochemical reactions using the nanofabricated electrochemical liquid cell was also presented.
The captured dynamic electrochemical reactions using the fabricated electrochemical liquid cells were also demonstrated for many mechanism studies, such as lithium dendritic growth and solid electrolyte interface (SEI) formation, electrochemical alloying and de-alloying of gold anodes, electrochemical lithiation and delithiation of molybdenum disulphide nanosheets. This technique opens a venue by which to look inside the electrochemistry at an unprecedented level, thus improving electrode design for reducing short-circuit failure and improving the performance of lithium-ion batteries.
How does this method differ from existing workflow using commercial liquid cells? What benefits does this bring?
If comparing our developed technology and commercial technology for the fabrication of electrochemical liquid cells, the differences are reflected in the growth process of silicon nitride and the spacer. The electrochemical liquid cell designed by our nanofabrication method possesses thinner SiNx imaging windows (35 nm) than commercial ones (50 nm).
It also possesses a thinner liquid layer (150 nm) created by a thin indium spacer in contrast to that of commercial products (1,000 nm) created by an O-ring. The thinner SiNx imaging windows and thinner liquid layer ensure that our fabricated liquid cell can capture electrochemical reactions with better TEM spatial resolution than the commercial ones.
Are there any electrochemical systems where this technology that would be especially applicable?
In our previous work, several electrochemical systems were studied by in-situ TEM observation using our fabricated liquid cell, such as system I (2 gold electrodes, commercial LiPF6/EC/DEC electrolyte), system II (titanium electrode/MoS2 electrode, commercial LiPF6/EC/DEC electrolyte), and system III (2 titanium electrodes, NaPF6/PC electrolyte).
All two electrode electrochemical systems, such as lithium-ion batteries and sodium-ion batteries, are suitable for this technology.
What properties of silicon nitride make it especially suitable for use in the imaging window?
Silicon nitride is an ideal window material due to its high hardness, good electron transmittance, thin layer thickness, and strong corrosion resistance.
High hardness, for example, helps to avoid window breakage during use, even under very thin thickness, while good electron transmittance can ensure the high imaging resolution of the TEM.
Strong corrosion resistance can prevent it from being corroded by the electrolyte during the electrochemical reactions.
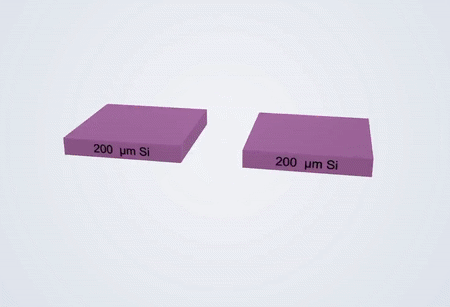
Fabrication process of the electrochemical liquid cell. (© Yang, R. et al. https://www.nature.com/articles/s41596-022-00762-y)
Could any other materials be used as the window instead of silicon nitride?
Graphene is an alternative window material for liquid cells beyond silicon nitride. The thin nature of the graphene window allows a high resolution for the liquid cell TEM. However, integrating electrodes into graphene liquid cells is challenging. Therefore, graphene windows are currently difficult for the fabrication of electrochemical liquid cells.
During fabrication, did the team encounter any problems, such as cell leakages? How were these resolved?
We have indeed encountered some problems in the manufacturing process. These problems and their solutions have been summarized in the Table 1 of our recent protocol published in Nature Protocols (Nat. Protoc. 2023, 18, 555-578). Among them, cell leakage is a common problem, which is normally caused by the deficient epoxy coating on the side of the liquid cell.
The solution to this problem is to carefully check the coating on the sides of the liquid cell by using an optical microscope to ensure that there are no holes on every side of the liquid cell.
How does the resolution of these cells compare to that of solid-state cells?
In our opinion, the resolution of our liquid cell is comparable with solid-state cells. However, liquid cell battery reactions are more representative of the real lithium ion and sodium ion battery reactions. Since the active electrode materials are immersed in a liquid electrolyte, full contact between the electrolyte and every side of the particles is ensured.
However, in in-situ solid-state cells, the solid electrolyte is not representative of the real solid electrolyte. Normally, the solid electrolyte used in in-situ solid-state cells are lithium oxide and ionic liquid, but in real solid-state cells, the solid electrolyte uses other materials rather than lithium oxide and ionic liquid.
Are there any challenges that remain regarding liquid cells that prevent a higher resolution from being achieved?
Indeed, reducing the thickness of silicon nitride can increase its electron transmittance, thus increasing the TEM imaging resolution. However, with the decrease in silicon nitride thickness, its mechanical strength will also decrease. Therefore, the thickness of silicon nitride should be greater than 35 nm to prevent breakage. This limits the further improvement of the imaging resolution by using this liquid cell.
Finding window materials with higher mechanical strength and better electron transmittance than silicon nitride is an effective way to improve the imaging resolution of the liquid cell.
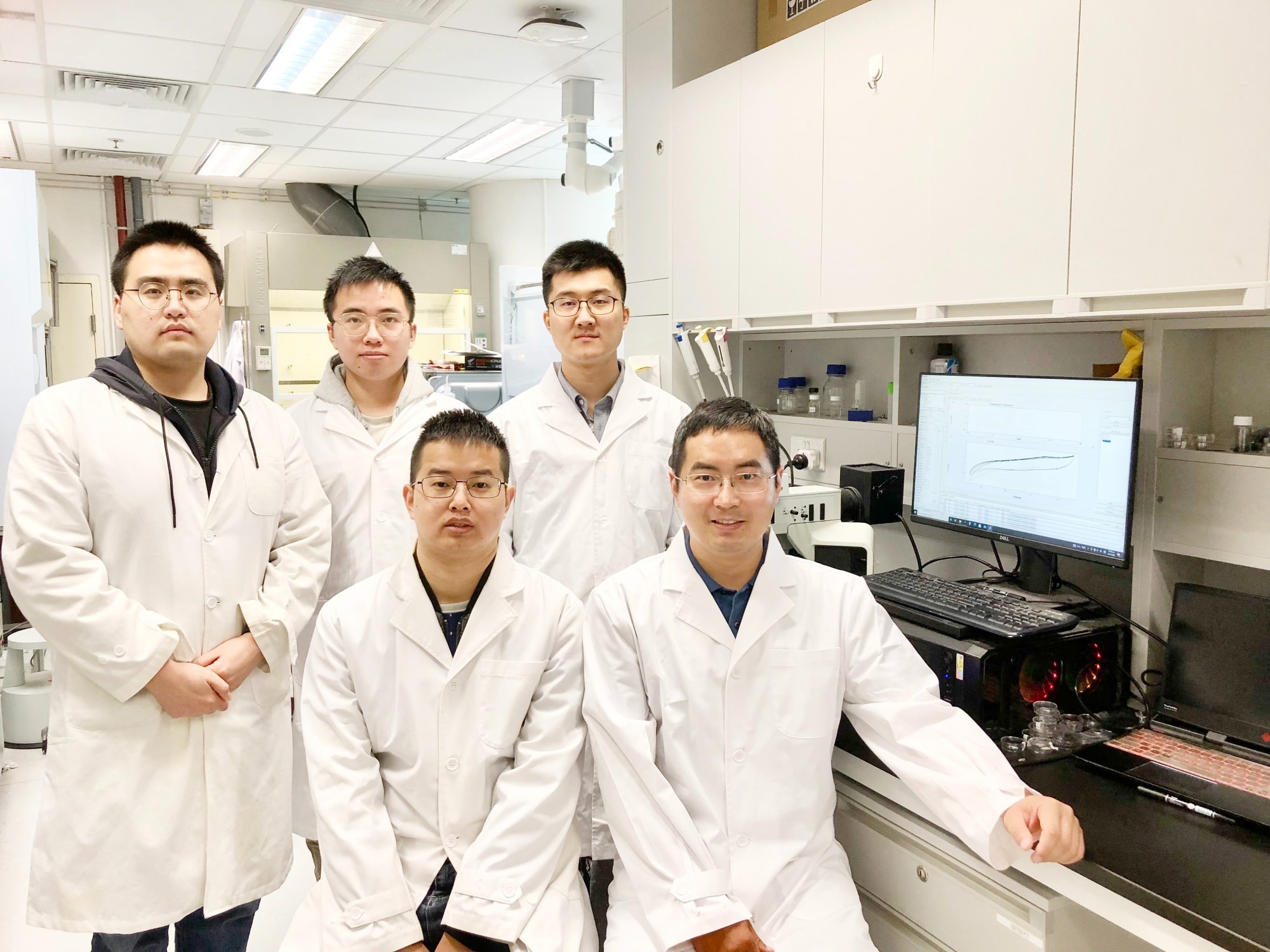
Dr. Zeng Zhiyuan (front row, right) and his research group from the Department of Materials Science and Engineering at City University of Hong Kong. (© City University of Hong Kong)
What are the next steps for this research?
In the next step, we are interested in extending this research in two directions. The first direction is on-demand fabrication of a series of structurally similar but functionally diverse electrochemical TEM liquid cells for the in-situ study of different electrochemical reactions. To achieve this goal, we need to adjust the types of deposited electrodes and the encapsulated liquid electrolytes as needed.
The second exciting direction is to extend this technology to manufacture electrochemical liquid cells for other in-situ characterization technologies beyond in-situ TEM, such as in-situ X-Ray absorption spectroscopy, in-situ X-Ray photoelectron spectroscopy, and in-situ X-Ray diffraction.
About Dr. Zhiyuan Zeng
Dr. Zhiyuan Zeng received his BSc, MPhil and Ph.D. degrees all in Material Science & Engineering from Central South University, Zhejiang University and Nanyang Technological University in 2006, 2008 and 2013, respectively. He continued his postdoc training at Lawrence Berkeley National Laboratory (2013-2017). Then he jumped into industry in Silicon Valley working for Applied Materials Inc. as a Senior Process Engineer (2017-2019).
Dr. Zeng joined CityU-MSE in 2019. His research interests are using battery intercalation strategy, in-situ liquid phase TEM to investigate TMDs and TMOs, which can be used for energy and environmental applications. He has published 111 SCI papers (72 IF>10) with a total citation of 18360 + times and H-index 53 (google scholar); 21 Papers were listed as ESI Highly Cited Papers, which include Nat. Mater., Nat. Protoc., Nat. Synth., Nat. Commun., Matter, Adv. Mater., Angew. Chem. Int. Ed., Nano Lett., etc.
He has been listed as the Highly Cited Researcher (Top 1%, Clarivate Analytics) in 2022, 2020 and 2018. He has been listed as the World's Top 2% Scientists in Nanosci. & Nanotechnol. in years 2022, 2021 and 2020 (by Stanford University). Dr. Zeng's other awards include the Rising Star by Advanced Materials (2022) and Small (2022), the Emerging Investigator by Chemical Communications (2021) and Journal of Materials Chemistry A (2020), the Early Career Scheme award by Research Grants Council of Hong Kong (2019).