From De GruyterReviewed by Louis CastelOct 11 2023
In this interview, AzoNano speaks to Professor Moti Segev and Professor Vlad Shalaev, who made surprising discoveries about photonic time crystals that challenge existing research and theories.
Could you provide an overview of the groundbreaking research published in the Nanophotonics Journal regarding photonic time crystals and their implications? What were the researchers hoping to achieve? Why is this area of study so intriguing?
Moti Segev:
As part of our research, we discussed photonic time crystals, which are materials that change their properties rapidly and significantly. The challenge we faced was changing the refractive index within a single optical cycle in the optical or near-infrared frequency regime to couple it with light-matter interactions.
To make it relevant for optical frequencies, we needed to change the refractive index by a large amount, approximately 0.1 or more, within one cycle, which spans only a few femtoseconds. This abrupt change results in time-refracted and time-reflected waves, the latter being a unique consequence of a temporal interface.
Traditional nonlinear optics were unable to achieve this level of change, so we explored alternative mechanisms, particularly transparent conducting oxides. With these materials, we managed to achieve the desired index change within just five femtoseconds and by 0.1 to 0.2, which was a significant breakthrough. Transparent conducting oxides show such strong and ultrafast responses when dielectric permittivity Epsilon is Near-Zero (so-called ENZ materials), resulting in anomalously small refractive indices.
Despite skepticism and claims of impossibility due to concerns about heat generation, we remained hopeful and pursued our vision. We were determined to push the boundaries of what was thought possible, and it paid off in the end.
Vlad Shalaev:
There was indeed skepticism regarding the material’s ability to respond rapidly and recover after each light cycle, a necessity for photonic time crystals. Surprisingly, our observations showed that the material not only responded within the cycle duration but also relaxed to its initial state within the same timeframe.
These observations contradicted the previous predictions and hinted at some kind of light-induced process, although the precise mechanism is still not fully understood. However, this uncertainty is part of the excitement in physics and presents an opportunity for discoveries.
Can you explain in simple terms the main hypothesis of the experiment and the specific behavior of photonic time crystals that the researchers were trying to observe? Was the initial experiment to try and disprove that this was impossible?
Moti Segev:
To create a photonic time crystal, we needed to periodically change the refractive index over a single cycle significantly. Traditional nonlinear optics mechanisms in solids, such as second harmonic generation, rely on one ground state and virtual energy levels. However, this approach was not viable here as it was too weak: the nonlinear change in the macroscopic polarization of the medium is orders-of-magnitude too weak.
Instead, we adopted a different strategy, focusing on altering the plasma frequency through the use of transparent conducting oxides, effectively modifying the kinetic energy of electrons. This alteration in plasma frequency depended on the photon flux of a laser pulse that brings electrons from the bottom of the conduction band to considerably higher energies.
The predictions that such a process would not work revolved around the relaxation of the high-energy electrons back to the bottom of the conduction band, which was thought to be electrons descending and converting their energy into heat via interactions with lattice vibrations (phonons). This process in semiconductors takes around 200 femtoseconds, at least. This conversion of the kinetic energy (of the electrons) into heat raised concerns that the material would eventually break due to the repeated energy-to-heat conversion.
The allure of the unknown and the belief that it was possible was what motivated us to embark on this experimental journey. We remained hopeful, and it proved worthwhile as we demonstrated it could be accomplished. There is more work still to be done, but it is an enabling technology that has the potential to open up new possibilities.
Vlad Shalaev:
Surprisingly, we experimentally achieved a significant change in refractive index (a few percent) at single-cycle, 5-6 femtosecond timescales, which is remarkable. But it is not just about demonstrating what is possible but about entering new realms of light-matter interaction, offering tremendous research opportunities.
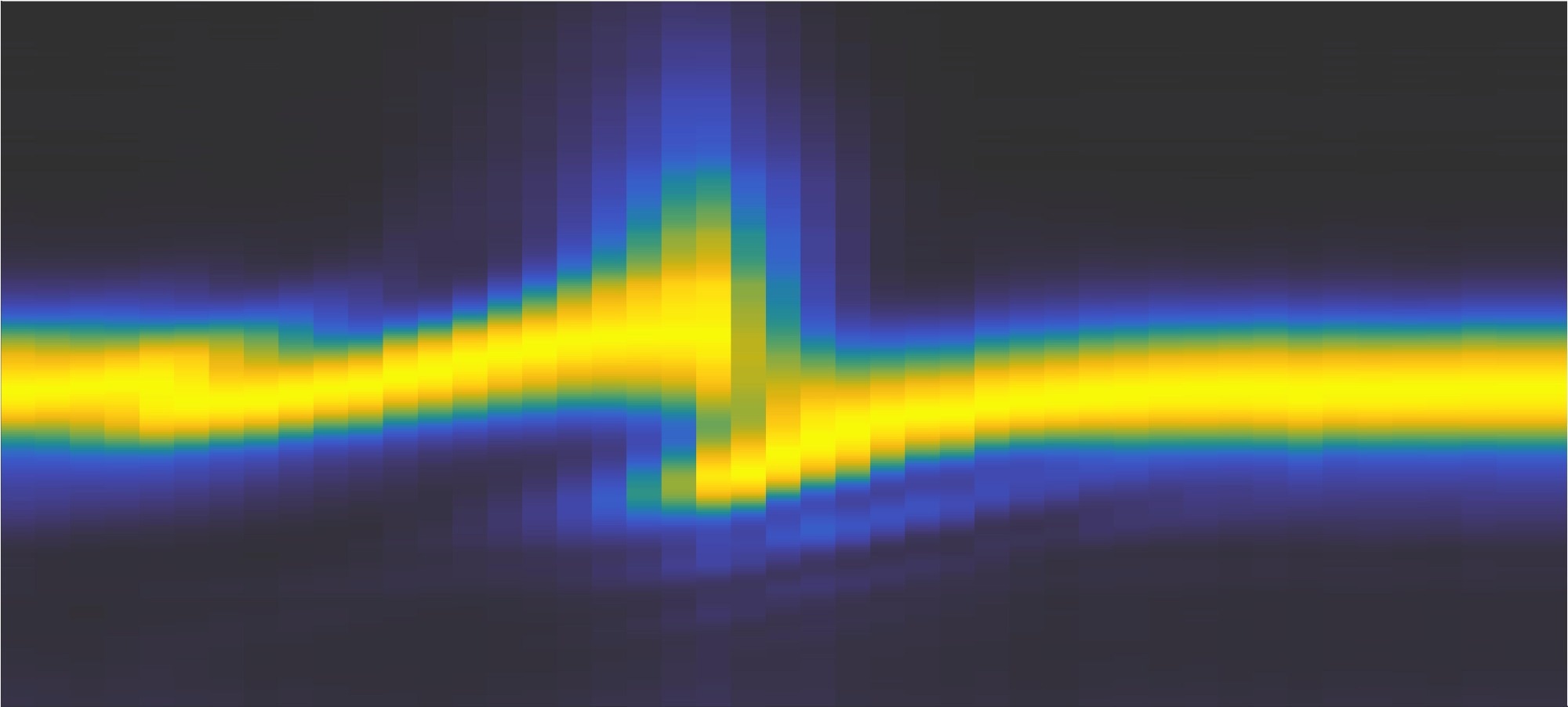
Image credits: De Gruyter
Could you help us to understand how these shifts were induced and controlled during the experiment?
Moti Segev:
To understand how these shifts were induced and controlled in our experiment, we utilized powerful lasers. First, we modified the material’s effective index with one laser pulse of 5-6 femtoseconds duration, and then used another laser pulse at 1500 nm near-infrared to sample the material’s response. Some of the key findings are outlined below:
- When the refractive index increased, the spectrum shifted to the red. This shift occurs due to conservation of momentum: the refractive index’s rise causes a corresponding decrease in frequency, resulting in a redshift in the spectrum.
- We monitored the power that was transmitted and reflected through the material.
- What stood out most was the spectrum shift behavior. When the refractive index increased significantly, the spectrum shifted to the red (lower frequency). Subsequently, as the material relaxed, the spectrum shifted to the blue (higher frequency). Interestingly, we observed that the rates of red and blue shifts were comparable. While we have a good model explaining the red shift, the rapid blue shift, where the effective index quickly returned to its original value, remains unexplained to this day. We have some ideas, but a definitive model has yet to be established.
Were there any surprising or unexpected findings during the experiment that challenged your initial assumptions or theories?
Moti Segev:
The astonishingly fast relaxation was the biggest surprise for me. I was not too shocked when the material did not explode. Frankly, I had my doubts about the papers suggesting such an outcome.
Vlad Shalaev:
I was concerned that some of the previous theoretical papers indicated that we might not be able to observe the single-cycle variations in refractive index required for photonic time crystals. Our experimental observations however clearly indicated that the needed fast changes in index can indeed occur, even though we don’t have yet complete explanation for this.
Similar to what Moti said, I found the system’s rapid return to its original state, including its refractive index, truly astonishing. This phenomenon is the key to enabling photonic time crystals, which we hope to demonstrate in the future under new light-matter interaction conditions. There is still some mystery here that we are yet to fully understand, which goes against ‘conventional’ theoretical calculations, but this opens up exciting opportunities for us in the future.
How do you think the findings are going to advance technology and research in the future?
Moti Segev:
Our findings have the potential to advance technology significantly. By harnessing these concepts, we can create photonic time crystals, opening doors to new radiation sources, signal amplification, and more.
Additionally, these ideas could lead to innovative resources for quantum cluster states and entangled photons. This field of coherent quantum light generation is still in its early stages, with most sources relying on spontaneous parametric down conversion (SPDC). While there are numerous ideas, success is uncertain, but there is a growing community working on it.
Vlad Shalaev:
From a broader perspective, our research suggests entirely new light-matter interactions, particularly related to the so-called band gaps.
Conventional photonic crystals, pioneered by Eli Yablonovitch, introduced truly novel concepts like inhibited spontaneous emission by creating band gaps in the frequency domain through the periodic modulation of dielectric permittivity in space. However, we are now shifting from the spatial domain to the time domain, periodically modulating material properties in time, which enbales exciting and intriguing phenomena such as time reflection and time refraction. The interference in multiple time reflection leads in turn to the formation of gaps in the momentum space, which are distinct from the frequency-based band gaps, offering intriguing possibilities for novel classical and quantum behaviour of light.
As Moti mentioned, this discovery can make possible the exponential amplification of light emission enabling new type of lasers, amplified entangled states, and facilitate the design of multi-photon cluster states, among other applications. Our next challenge is to demonstrate time reflection in the optical domain and then move on to the demonstration of photonic time crystals.
What makes photonic time crystals different from traditional photonic crystals?
Moti Segev:
In photonic time crystals, the system differs significantly from traditional photonic crystals. While photonic crystals have a band gap in frequency, preventing spontaneous emission within that range and enabling applications like thresholdless lasers, photonic time crystals operate differently. In photonic time crystals, energy conservation is replaced by momentum conservation that holds even during the process of modulation of the refractive index.
This modulation amplifies light within the momentum band gap, irrespective of its frequency, drawing energy from the modulation itself. This distinction is quite exciting, as it focuses on the amplification of signals rather than the inhibition of emission, making it distinct from traditional photonic crystals.
Vlad Shalaev:
The core difference between photonic time crystals and traditional photonic crystals lies in the concept of band gaps. Photonic crystals conventionally manipulate material properties in space, creating band gaps in the frequency domain. This manipulation has led to various applications such as optical cavities, waveguides, and control of spontaneous emission. However, photonic time crystals operate by modulating material properties in the time domain, resulting in a band gap in the momentum space.
This distinction is crucial because it introduces fundamentally different properties compared to traditional photonic crystals, especially when transitioning from electrons to photons.
Why is it important to extend the study of photonic time crystals to other parts of the electromagnetic spectrum? How might this expansion benefit the field of optics?
Moti Segev:
In the realm of electromagnetism, achieving effective light-matter coupling can be challenging. The most efficient coupling occurs through electronic levels, which is why the visible and infrared spectra are particularly crucial.
In contrast, when attempting to couple light at different frequencies, such as microwaves, most of the interaction behaves like an antenna. However, in the case of microwaves coupled with light-matter interactions, the energy is typically dissipated.
In the visible spectrum, light drives electrons from lower energy bands to higher ones. This differs significantly from the microwave scenario. This underscores the importance of bridging these interactions into the optical domain.
How do you foresee photonic time crystals contributing to the future of light-based technologies?
Vlad Shalaev:
Our experimental observations have revealed the dynamics that surpass traditional theories like the Drude model, Ohm’s law, or the two-temperature model. These observations defy conventional explanations, signifying a regime where events occur faster than the decoherence processes associated with collisions in materials.
This opens the door to witnessing new quantum phenomena. We find ourselves in a realm where processes unfold more rapidly than the standard relaxation processes that typically disrupt classical and, most importantly, quantum coherence and entanglement needed to enable the unique quantum properties.
This unparalleled regime could lead to novel interactions and phenomena, as it operates on a timescale far faster than what conventional relaxation theories can accommodate. We anticipate shedding new light on such meta-interactions and observing genuinely unprecedented phenomena.
Now that you have published this groundbreaking research, what is the next step? What do you intend to do with this research?
Moti Segev:
Our immediate goal is to observe time reflections and subsequently create time crystals.
Vlad Shalaev:
We have already achieved time refraction, and our next challenge is time reflection and eventually photonic time crystals. To accomplish this, we will need to send several pulses periodically, enabling the creation of photonic time crystals through strong and ultrafast modulation in the time domain. That is our focus for the coming year.
About the speakers
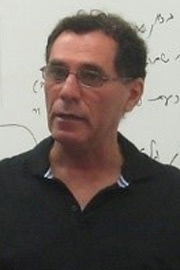
Moti Segev is the Robert J. Shillman Distinguished Professor of Physics and Electrical Engineering, at the Technion, Israel. He received his BSc and PhD from the Technion in 1985 and 1990. After a postdoc at Caltech, he joined Princeton as Assistant Professor (1994), becoming Associate Professor in 1997, and Professor in 1999. Subsequently, Moti went back to Israel, and in 2009 was appointed as Distinguished Professor. He won numerous international awards, among them the 2007 Quantum Electronics Prize of the EPS, the 2009 Max Born Award of the OSA, and the 2014 Arthur Schawlow Prize of the APS. He is a Member of the Israel Academy of Sciences, and International Member of the National Academy of Science (USA), and of the American Academy of Arts and Sciences (AAAS). In 2014 he won the Israel Prize (highest honor in Israel) and in 2019 he has won the EMET Prize. However, above all his personal achievements, Moti takes pride in the success of his graduate students and postdocs, among them are currently 25 professors and many holding senior R&D positions in the industry.
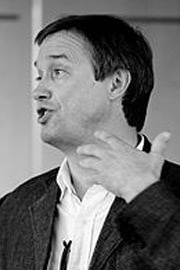
Vladimir M. Shalaev, Scientific Director for Nanophotonics at Birck Nanotechnology Center and Distinguished Professor of Electrical and Computer Engineering at Purdue University, specializes in nanophotonics, plasmonics, optical metamaterials and quantum photonics. Prof. Shalaev has received several awards for his research, including the APS Frank Isakson Prize for Optical Effects in Solids, the Max Born Award of the Optical Society of America for his pioneering contributions to the field of optical metamaterials, the Willis E. Lamb Award for Laser Science and Quantum Optics, IEEE Photonics Society William Streifer Scientific Achievement Award, Rolf Landauer medal of the ETOPIM (Electrical, Transport and Optical Properties of Inhomogeneous Media) International Association, the UNESCO Medal for the development of nanosciences and nanotechnologies, and the OSA and SPIE Goodman Book Writing Award. He is a Fellow of the IEEE, APS, SPIE, MRS and OSA.
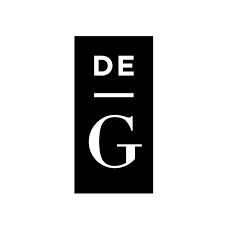
To read the full article, please visit De Gruyter.
This information has been sourced, reviewed and adapted from materials provided by De Gruyter.
For more information on this source, please visit De Gruyter.
Disclaimer: The views expressed here are those of the interviewee and do not necessarily represent the views of AZoM.com Limited (T/A) AZoNetwork, the owner and operator of this website. This disclaimer forms part of the Terms and Conditions of use of this website.