While Raman spectroscopy has been hugely successful in a number of domains of chemistry and materials science,1-3 one of the main challenges with performing Raman measurements is the inherently weak nature of the Raman signal.
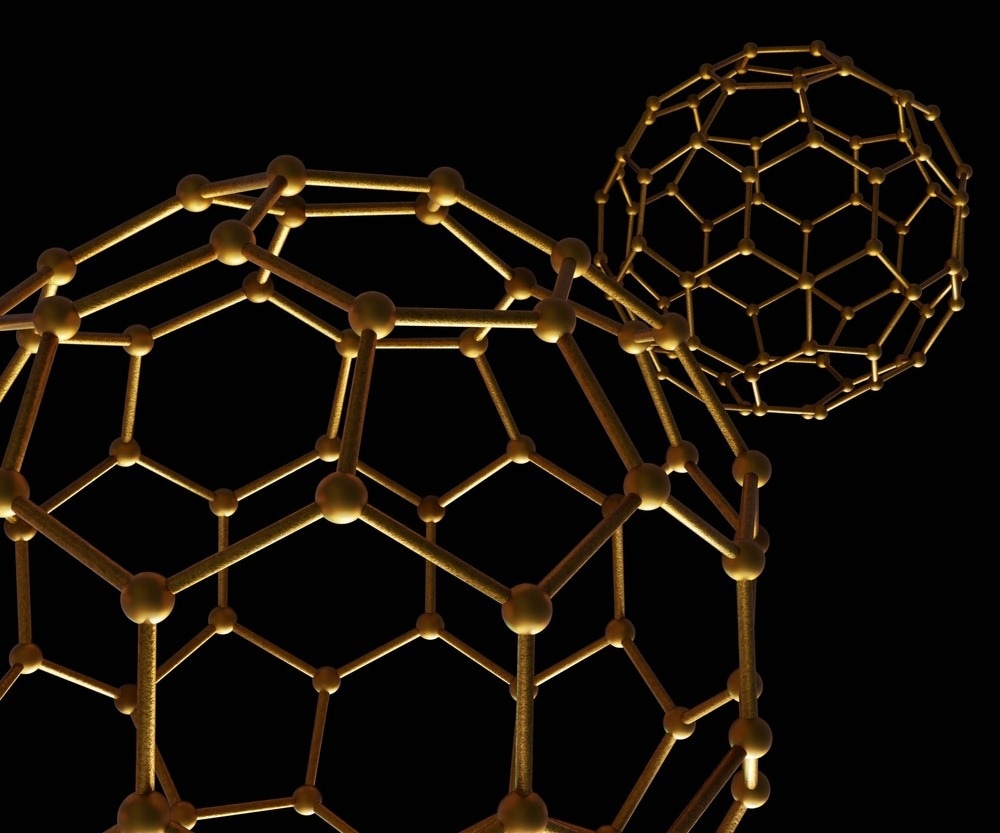
Image Credit: Love Employee/Shutterstock.com
The intensity of Raman scattering scales with the inverse fourth power of the incident wavelength used in the experiment; shorter excitation wavelengths are key to achieving better signal levels.
However, in practical terms for measurements, shorter wavelengths have a greater probability of exciting electronic transitions, which can potentially create a large, broadband fluorescence background that can obscure the relatively weak Raman signatures.4
One way of improving Raman experiments is to use a higher repetition rate or more intense laser sources. However, there is a greater risk of sample damage with increasing photon dose.5 Another approach is to make use of plasmonic effects to enhance the Raman signal, which is one of the basic principles behind techniques such as surface-enhanced Raman spectroscopy (SERS).
Gold and silver nanoparticles are an ideal choice of material for exploiting plasmonic enhancement in Raman spectroscopy due to their own strong plasmonic effects and the tunability of these properties through control over the size of the nanoparticles produced.6
Plasmonic Enhancement
Plasmonics concerns the manipulation of optical frequencies using metal-dielectric interfaces on the nanometer length scale. Plasmonic enhancement is a type of field enhancement effect that often occurs close to the surface of metals, where the collective motion of the particles near the surface of the metal can drive processes such as charge localization.7
For Raman spectroscopy, gold nanoparticles can be used to drive a significant field enhancement when the nanoparticles are resonant with the incident light. Gold nanoparticles have very high extinction coefficients, a tunable and broad absorption spectrum and are generally very chemically stable with good biocompatibility, so can be used in a number of environments and with a variety of sample types, including biomaterials.
Techniques with SERS, that rely on the plasmonic enhancement to improve the Raman signal work by using a SERS substrate – very often a functionalized gold nanoparticle – that is tethered to the molecule or substrate of interest and drives the amplification of the local plasmonic resonances.8 SERS can theoretically reach enhancement levels up to 1010 to 1011 times.
Spectroscopic Applications
A large variety of tuned gold nanoparticles have now been developed over the last few years since the original observation of the SERS effect in 1974.8 The improved range and availability of plasmonic enhance has meant SERS has been highly successful in a number of research areas, including sensing, materials science and the biosciences.
The inherently low signal levels of Raman spectroscopy mean that the limit of detection of Raman spectroscopy is such that it is challenging to make measurements on dilute or low-concentration samples. One of the key advantages of SERS is improvements in the limits of detection of Raman spectroscopy to the point where it is now possible to measure samples at the single molecule level.9
Stronger signal levels can also be exploited for reduced acquisition times, so meaningful spectra can be acquired on less sensitive instruments. Handheld devices are often miniaturized versions of laboratory instruments, but miniaturization often faces the challenges that, due to constraints on the footprint and power draw, it can be hard to achieve the same collection efficiency with the detection optics or the same power output of the source laser.
Many research groups have been developing SERS substrates specifically for compatibility with handheld devices, including using gold nanoshells for their excellent resonant enhancement effects.10
Tuning the optical absorption maxima to match the often-fixed laser wavelengths in handheld instruments is one consideration when developing SERS substrates for portable instruments, as well as making compounds that have sufficient long-term stability for field measurements and easy preparation that do not require the use of specialized equipment for sample preparation.
Gold nanoparticles and their plasmonic properties have been particularly well-exploited in the life sciences, where the biocompatibility of gold gives it an excellent advantage over many other types of plasmonic substrates. Plasmon-enhanced fluorescence and SERS have proved invaluable methods in biological imaging.11
The small size of gold nanoparticles makes them highly mobile in tissues and organs, and so researchers have been able to exploit this to measure flow rates and the motion of particle motor proteins along their native microtubulue environments.11
Capturing biomolecular dynamics and mechanics in action with the high spatial resolutions achievable with Raman imaging has led to a wealth of understanding about how proteins move and interact with each other and how the forces between cells play a role in transport mechanisms in the body.
Raman spectroscopy has been one of the dominant workhorse techniques in the analytical sciences, and the ability to exploit materials like gold nanoparticles to enhance signal levels has broadened the scope of the substances and measurement conditions that can be exploited with Raman-based methods.
References and Further Reading
Shekhirev, M., et al. (2021) Characterization of MXenes at every step , from their precursors to single flakes and assembled films. Progress in Materials Science, 120, p.100757. doi.org/10.1016/j.pmatsci.2020.100757
Hollon, T. C., et al. (2020) Near real-time intraoperative brain tumor diagnosis using stimulated Raman histology and deep neural networks. Nature Medicine, 26(January). doi.org/10.1038/s41591-019-0715-9
Neyman, P. J., et al. (2017) Free electron lasers in 2017. Proceedings of the 38th International Free-Electron Laser Conference, FEL 2017, pp.204–209. doi.org/10.18429/JACoW-FEL2017-MOP066
Jones, R. R., et al. (2019) Raman Techniques : Fundamentals and Frontiers. Nanoscale Research Letters, 14, p.231. doi.org/10.1186/s11671-019-3039-2
Fu, Y., et al. (2006) Characterization of photodamage in coherent anti-Stokes Raman scattering microscopy. Optics Express, 14(9), p.3942. doi.org/10.1364/oe.14.003942
Aldosari, F. M. M. (2022) Characterization of Labeled Gold Nanoparticles for Surface-Enhanced Raman Scattering. Molecules, 27(3). doi.org/10.3390/molecules27030892
Yu, H., et al. (2019) Plasmon-enhanced light–matter interactions and applications. Npj Computational Materials, 5(1), pp.1–14. doi.org/10.1038/s41524-019-0184-1
Sharma, B., et al. (2012) SERS: Materials, applications, and the future. Materials Today, 15(1–2), pp.16–25. doi.org/10.1016/S1369-7021(12)70017-2
Mao, P., et al. (2018) Broadband single molecule SERS detection designed by warped optical spaces. Nature Communications, 9(1), pp.1–8. doi.org/10.1038/s41467-018-07869-5
Kearns, H., et al. (2017) Sensitive sers nanotags for use with a hand-held 1064 nm raman spectrometer. Royal Society Open Science, 4(7). doi.org/10.1098/rsos.170422
Wu, Y., et al. (2019) Gold nanoparticles in biological optical imaging. Nano Today, 24, pp.120–140. doi.org/10.1016/j.nantod.2018.12.006
Disclaimer: The views expressed here are those of the author expressed in their private capacity and do not necessarily represent the views of AZoM.com Limited T/A AZoNetwork the owner and operator of this website. This disclaimer forms part of the Terms and conditions of use of this website.