A fuel cell is a device that converts fuel directly into electricity in an electrochemical reaction. This contrasts with most methods of generating electricity, which use the heat from burning fuel to generate electricity mechanically.
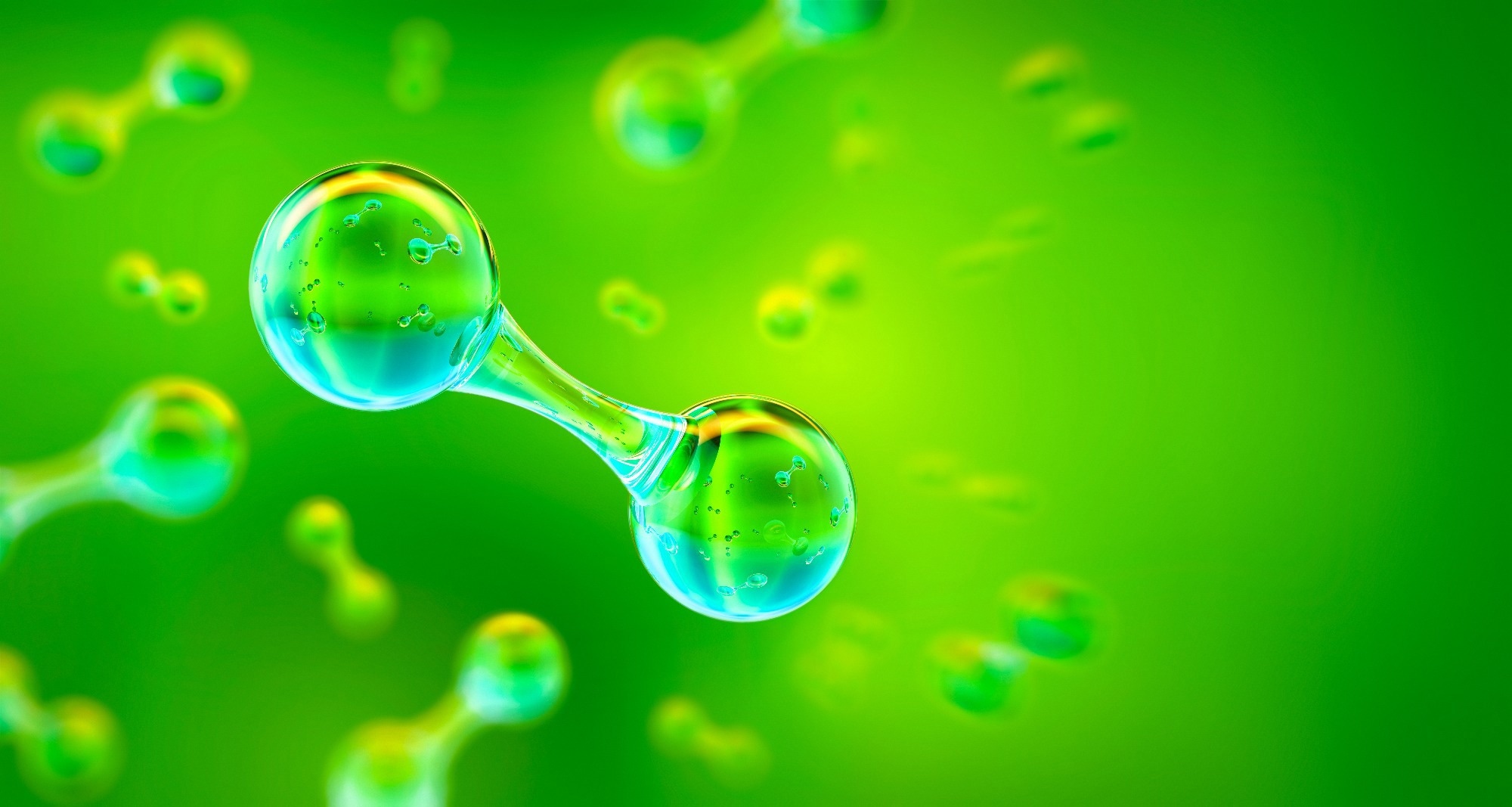
Image Credit: Corona Borealis Studio/Shutterstock.com
Fuel cells are an incredibly efficient power source. They can operate on various fuels and are scalable from small devices, like laptops, to large installations powering data centers.
Hydrogen, a preferred fuel for fuel cells, is a clean and potentially sustainable energy vector that has gained significant attention as a key enabler for a multisectoral transition towards a renewable energy source-based low-carbon economy. Hydrogen can be produced from electricity through electrolysis and then reconverted into electricity using fuel cells with relatively high efficiency.1
However, many limitations prevent fuel cells from reaching widespread commercial use. Hydrogen is costly to produce sustainably and difficult to store, while other fuel options can lead to fouling of the electrodes. Additionally, fuel cells often rely on expensive materials, such as platinum, for electrode catalysts.
The most efficient fuel cells operate at high temperatures, but this can reduce their lifespan due to corrosion of internal components. Recent advancements in nanotechnology offer potential solutions to these issues. Research into nanomaterials has shown promise in making fuel cells more affordable, lighter, and efficient.
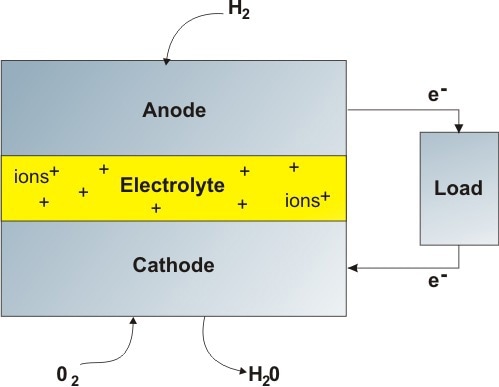
Figure 1. Schematic of a hydrogen fuel cell. The two electrodes act as catalysts for the chemical reaction, as well as passing charged particles across the electrolyte. The electrolyte is usually a conducting solid ceramic, aqueous solution, or molten phosphoric acid.
Nanotechnology for Fuel Cell Catalysts
The catalytic electrodes in fuel cells are typically made from platinum, a material that is both expensive and scarce. Research has shown that using platinum nanoparticles rather than a solid platinum surface increases efficiency and reduces the amount of platinum required.
One promising approach to further improve this technology is to support platinum nanoparticles on a porous surface, such as activated carbon or nanostructures like carbon nanotubes or nanowalls. This would further increase the accessibility of the platinum surfaces, decreasing the amount of the expensive metal needed to make an effective catalytic electrode.
Using nanomaterials in fuel cell electrodes substantially increases the catalytic surface area, reduces the device size, and extends life cycles, enabling next-generation fuel cell technology. Despite these advantages, it remains essential to address human health and environmental considerations in the selection and design of these nanomaterials.2,3
New Catalyst Developments
Recent technological advances have led to the development of novel materials, including platinum group metal-free (PGM-free) catalysts, for fuel cell catalysis and ion transport. This has led to reliable and efficient fuel cell performance.2,4
Alloying platinum with transition metals effectively enhances electrocatalytic activity while reducing platinum use and overall cost. For instance, one-dimensional bunched platinum-nickel nanocages developed for oxygen reduction reactions (ORRs) in fuel cells demonstrate specific and mass activities that are 14.3 and 16.8 times higher, respectively, than traditional platinum/carbon catalysts.4
Polymer electrolyte membrane fuel cells (PEMFCs) and direct methanol fuel cells (DMFCs), which use platinum alloys in their anodes and cathodes, achieve electrical efficiencies between 40 % and 60 %. PEMFCs provide advantages like compact structure, high power density, and rapid startup.4
Among the PGM-free catalysts, the metal and nitrogen co-doped carbon (M-N-C) catalysts, where M is the d-transition element of the fourth period, are the best alternatives to platinum-based catalysts. For instance, single atomic iron-rich catalysts synthesized using a novel three-dimensional (3D) polyaniline hydrogel approach demonstrate excellent activity and stability, achieving a half-wave potential of 0.83 V—one of the top performances for PGM-free catalysts.4
The use of metal-organic frameworks (MOFs) for M-N-C synthesis offers additional benefits due to their unique properties and simpler synthesis process. Imidazole-type ligands, typically 2-methylimidazole combined with metal like zinc, are mostly used in M-N-C catalyst synthesis.4
For instance, 0.88 V half-wave potential can be achieved using MOF-derived iron-N-C, which is 14 mV higher than platinum catalysts. Additionally, the crumpled catalyst with highly dispersed iron atoms embedded within graphitic layers possesses long-term durability in alkaline media, excellent catalytic activity, and a direct 4e- reaction path, eliminating hydrogen peroxide production.4
Enhanced Nanostructures
The use of hybrid nanomaterials as catalysts in fuel cells improves efficiency and durability.
For instance, platinum (copper) nanoparticles supported on multi-walled carbon nanotubes (MWCNTs) (Pt(Cu)/MWCNTs) are well-suited for low-temperature fuel cells. These nanostructures demonstrate a high tolerance to carbon monoxide, enhanced mass and specific activity, and greater resistance to corrosion compared to traditional platinum/carbon cathode catalysts.4
Similarly, nitrogen-doped carbon nanotubes combined with reduced graphene oxide (rGO) nanosheet hybrids can be used as platinum-free electrocatalysts for microbial fuel cell (MFC) cathodic oxygen reduction. This hybrid electrocatalyst achieves a half-wave potential of 0.859 V with good stability, making it a promising cathodic catalyst for MFCs. It can also deliver enhanced cathodic oxygen reduction and power generation.5
Self-cleaning Catalysts
Self-cleaning catalysts are emerging as valuable technology in fuel cells. They enable expanded fuel options and facilitate lower-temperature operations, which contribute to improved efficiency and durability.
Expanded Fuel Options: Biological fuel cells (BFCs) are a type of low-temperature fuel cell that convert waste biomass to electricity through enzymatic reactions (enzymatic fuel cells) or microbially catalyzed reactions (microbial fuel cells). They operate near ambient temperatures (15 ° C to 45° C), and reactions are biologically catalyzed using proteins, bacteria, or enzymes. The use of waste biomass makes them a sustainable option compared to other fuel cells.4
Lower Temperature Operations: PEMFCs and DMFCs typically operate at low temperatures, up to 100 °C. Catalysts that facilitate operations in these low-temperature fuel cells include platinum-ruthenium/single-walled carbon nanotubes (SWCNTs) or MWCNTs, platinum-rGO, palladium-iron/carbon, nitrogen-doped graphene oxide, and bimetallic-organic framework derived HC-5Co95Zn.4
Thin-Film Electrolyte Membranes
Thin-film electrolyte membranes, particularly those using advanced materials like perovskites and cutting-edge manufacturing techniques, are set to significantly enhance the performance and durability of solid oxide fuel cells.4,6,7
New Electrolyte Materials: The electrochemical performance of proton ceramic fuel cells (PCFCs) is significantly influenced by the morphological and compositional characteristics of the electrolyte materials. These fuel cells have high energy conversion efficiency and generate low carbon emissions at low operating temperatures.6
Electrolyte materials derived from cerate–zirconate ceramic perovskite-type oxides display exceptionally high proton conductivity in water- and hydrogen-containing atmospheres.6
Improved Manufacturing Techniques: Advanced methods allow for larger, more stable membrane films. For instance, zirconium dioxide applied via atomic layer deposition has been used as a catalyst for molten carbonate fuel cells operating at 600° C.4
Depositing metals and metal oxides onto surfaces using supercritical fluids (SCFs) has also gained significant attention due to the favorable properties of SCFs, including the absence of liquid waste, adjustable solvent power through pressure and temperature changes, and seamless miscibility with reactive gases like hydrogen or oxygen.7
In fuel cells, carbon-supported platinum electrocatalysts synthesized using supercritical deposition exhibit exceptional efficiency in hydrogen oxidation and oxygen reduction reactions.7
Applications and Commercialization
Fuel cells have a wide range of applications, from stationary power generation for off-grid and backup electricity to portable electronics due to their compact design. They are also increasingly used in aerospace and transportation as industries seek zero-emission solutions.8
Hydrogen fuel cells capable of generating megawatt-scale electricity provide a reliable, stable, and zero-emissions energy supply for warehouses, data centers, buildings, regional electric grids, and industrial sites. In the automotive sector, companies like Toyota and Honda are developing fuel cell vehicles with extended ranges compared to traditional battery electric vehicles.8
Fuel cells are also a critical component of carbon-neutral initiatives. By utilizing clean hydrogen, derived from renewable energy sources like solar and wind, fuel cells can generate electricity with zero emissions. This integration of fuel cells with renewable energy systems offers a promising pathway towards a sustainable and low-carbon future.9
Specifically, green hydrogen has the potential to support the integration of renewable energy sources into future energy systems as a carbon-neutral and versatile energy carrier produced from renewable electricity. Integrating green hydrogen can help address energy storage and supply intermittency challenges associated with renewables, contributing to a more stable and reliable energy system.9
In summary, advances in nanotechnology are transforming fuel cell technology by addressing challenges such as high costs, efficiency limitations, and restricted fuel options. By developing advanced nanomaterials for catalysts and membranes, researchers are paving the way for more efficient, durable, and sustainable fuel cell systems.
The Future of Hydrogen Production
References and Further Reading
- Falcone, PM., Hiete, M., Sapio, A. (2021). Hydrogen economy and sustainable development goals: Review and policy insights. Current Opinion in Green and Sustainable Chemistry. DOI: 10.1016/j.cogsc.2021.100506, https://www.sciencedirect.com/science/article/pii/S2452223621000626
- Karthik Pandiyan, G., Prabaharan, T. (2020). Implementation of nanotechnology in fuel cells. Materials Today: Proceedings. DOI: 10.1016/j.matpr.2020.01.368, https://www.sciencedirect.com/science/article/abs/pii/S2214785320304697
- Falinski, MM., Plata, DL., Chopra, SS., Theis, TL., Gilbertson, LM., Zimmerman, JB. (2018). A framework for sustainable nanomaterial selection and design based on performance, hazard, and economic considerations. Nature Nanotechnology. DOI: 10.1038/s41565-018-0120-4, https://www.nature.com/articles/s41565-018-0120-4
- Sajid, A., Pervaiz, E., Ali, H., Noor, T., Baig, MM. (2022). A perspective on development of fuel cell materials: Electrodes and electrolyte. International Journal of Energy Research. DOI: 10.1002/er.7635, https://onlinelibrary.wiley.com/doi/abs/10.1002/er.7635
- Du, Y. et al. (2019). Nitrogen-doped carbon nanotubes/reduced graphene oxide nanosheet hybrids towards enhanced cathodic oxygen reduction and power generation of microbial fuel cells. Nano Energy. DOI: 10.1016/j.nanoen.2019.05.001, https://www.sciencedirect.com/science/article/abs/pii/S2211285519304008
- Nur Syafkeena, MA., et al. (2022). Review on the preparation of electrolyte thin films based on cerate-zirconate oxides for electrochemical analysis of anode-supported proton ceramic fuel cells. Journal of Alloys and Compounds. DOI: 10.1016/j.jallcom.2022.165434, https://www.sciencedirect.com/science/article/abs/pii/S0925838822018254
- Escorcia-Díaz, D., García-Mora, S., Rendón-Castrillón, L., Ramírez-Carmona, M., Ocampo-López, C. (2023). Advancements in nanoparticle deposition techniques for diverse substrates: a review. Nanomaterials. DOI: 10.3390/nano13182586, https://www.mdpi.com/2079-4991/13/18/2586
- A. Qasem, NA., Q. Abdulrahman, GA. (2024). A Recent Comprehensive Review of Fuel Cells: History, Types, and Applications. International Journal of Energy Research. DOI: 10.1155/2024/7271748, https://onlinelibrary.wiley.com/doi/full/10.1155/2024/7271748
- Marouani, I., et al. (2023). Integration of Renewable-Energy-Based Green Hydrogen into the Energy Future. Processes. DOI:10.3390/pr11092685, https://www.mdpi.com/2227-9717/11/9/2685
- Gong, K., Du, F., Xia, Z., Durstock, M., Dai, L. (2009). Nitrogen-doped carbon nanotube arrays with high electrocatalytic activity for oxygen reduction. Science. DOI: 10.1126/science.1168049, https://www.science.org/doi/abs/10.1126/science.1168049
- Tsuchiya, M., Lai, B., Ramanathan, S. (2011). Scalable nanostructured membranes for solid-oxide fuel cells. Nature Nanotechnology. DOI: 10.1038/nnano.2011.43, https://www.nature.com/articles/nnano.2011.43
- Wang, S., Yu, D., Dai, L. (2011). Polyelectrolyte functionalized carbon nanotubes as efficient metal-free electrocatalysts for oxygen reduction. Journal of the American Chemical Society. DOI: 10.1021/ja1112904, https://pubs.acs.org/doi/abs/10.1021/ja1112904
Disclaimer: The views expressed here are those of the author expressed in their private capacity and do not necessarily represent the views of AZoM.com Limited T/A AZoNetwork the owner and operator of this website. This disclaimer forms part of the Terms and conditions of use of this website.