There are various methods in making CNTs and fullerenes. The first time scientists have observed fullerenes was after vaporizing graphite with a short-pulse, high-powered laser. Yet, this method is not advised because it’s not practical in creating large quantities. CNTs have existed for a longer period than when it was first observed. They may have been created during many carbon combustion and vapor deposition processes. But, electron microscopy during that time was not yet modern and can’t distinguish the CNTs from other forms of carbon.
The application of electric current across two carbonaceous electrodes in an inert gas atmosphere was the first method for creating CNTs and fullerenes in sensible quantities. The method is dubbed as plasma arcing, which implicates the evaporation of one electrode as cations trailed by the deposition at the other electrode. The process, which is plasma-based, is similar to the more familiar electroplating process in a liquid form. Fullerenes and CNTs are created by plasma arcing of carbonaceous materials, specifically graphite.
The fullerenes or nanotubes appear in the soot that is produced, while the CNTs are placed on the opposite electrode. Plasma arcing is another method of nanotube synthesis with cobalt with a 3 percent or greater concentration. As stated above, the nanotube product is a compact cathode deposit of rod like morphology.
But, when cobalt is added as a catalyst, the product’s nature evolves into a web with strands of 1 mm thickness. These stretch from the cathode to the walls of the reaction vessel. The mechanism wherein cobalt changes this process is still unknown but one possible answer is that such metals influence the local electric fields. Thus, the five-membered rings are formed.
Arc Method
The carbon arc discharge method, first utilized for creating C60 fullerenes, is one of the most common and easiest way to create CNTs, because it is simple. Yet, the technique creates a complicated mixture of components. It also requires additional purification to isolate the CNTs from the soot and the residual catalytic metals existing in the unpolished product.
.jpeg)
A Process Diagram for Arc Method CNT Synthesis
The method makes CNTs through arc-vaporization of two carbon rods situated end to end in a location that is filled with low pressure and inert gas. The discharge evaporates the surface of one of the carbon electrodes, and creates a tiny rod-shaped deposit on the opposing electrode. The production of CNTs in high harvest relies on the uniformity of the plasma arc, and the temperature of the deposit growing on the carbon electrode.
Hipco method is a type of arc method synthesis method achieved under high pressure and was established at Rice University to produce high quality single-walled carbon nanotubes (SWCNT) from the gas-phase reaction of iron carbonyl with high pressure carbon dioxide gas. People use iron pentacarbonyl to make iron nanoparticles that give a nucleation surface for carbon monoxide transformation into carbon during the development of the nanotubes. Synthesis creates high quality materials but in just small quantities and they’re not available to be distributed in the amounts needed commercially.
Laser Methods
Carbon nanotubes were first synthesized in 1996, with a dual-pulsed laser and produced yields of >70 wt% purity. Laser vaporization prepared samples of graphite rods with a 50:50 catalyst mixture of Nickel and Cobat at 1200 oC in flowing argon. It was then followed by heat treatment in a vacuum at 1000 oC to eradicate the C60 and other fullerenes. The first laser vaporization pulse was trailed by a succeeding pulse, to vaporize the target more consistently.
The utilization of two consecutive laser pulses reduces the amount of carbon collected as soot. The second laser pulse disbands the bigger particles ablated by the initial one. It then feeds them into the developing nanotube structure. The material made by this process is shown as a mat of “ropes”, about 10 to 20 nm in diameter and up to 100 µm or more in length.
Each rope contains a bundle of single-walled nanotubes, in parallel to a common axis. By having different growth temperature, the catalyst configuration and other process parameters, the average nanotube diameter and size alignment becomes diverse.
Arc-discharge and laser vaporization are presently the main methods for getting tiny quantities of high quality CNTs. Yet, the two methods face some problems. First off, they involve the evaporation of the carbon source. So, it’s unknown how to boost production to the industrial level using these methods. Second, vaporization methods grow CNTs in highly twisted forms, combined with unwanted forms of carbon and/or metal types. Hence, the CNTs made are hard to purify, gather and handle for developing nanotube-device architecture for useful implications.
Catalyzed Chemical Vapor Deposition
Undeniably, the most common method of carbon nanotubes synthesis is catalyzed chemical vapor deposition of hydrocarbons. It’s a traditional process used to create several carbon materials such as carbon fibers and filaments for over two decades.
Huge amounts of CNTs can be produced by catalytic CVD of acetylene over iron catalysts and Cobalt, which are supported on zeolite or silica. The carbon deposition activity is linked to the cobalt content of the catalyst. On the other hand, the CNTs’ discernment seems to be a role of the pH in the preparation of the catalyst.
Multi-walled nanotubes made on the carbon/zeolite catalyst contain fullerenes and bundles of single-walled nanotubes. Supported catalysts including cobalt, iron, and nickel, which contain either a mixture of metals or a single metal, prompt the development of isolated single-walled nanotubes or single-walled nanotubes bundles in the ethylene atmosphere.
.jpeg)
A Process Diagram for Catalyzed Chemical Vapor Deposition CNT Synthesis
The creation of single-walled nanotubes and double-walled CNTs on molybdenum and molybdenum-iron alloy catalysts has also been shown. Methane has been utilized as a carbon source. Specifically, it has been used to get ‘nanotube chips’ comprising of isolated single-walled nanotubes at controlled areas.
Ball Milling
Ball milling and subsequent annealing is a simple method for creating CNTs. Despite the fact that mechanical attrition of this form can lead to fully nano porous microstructures, it was not until a few years back that CNTs of carbon and boron nitride were made from these powders by a method called thermal annealing.
Importantly, the method involves the placement of graphite powder into a stainless steel container with four hardened steel balls. The container is removed and argon is introduced. The milling is demonstrated at room temperature for about 150 hours long. After the milling process, the powder is hardened under an inert gas flow at temperatures of about 1400 oC for six hours. The mechanism of the method is unclear, but it is believed that the ball milling process forms nanotube nuclei, and the annealing process triggers the growth of nanotubes. Studies have shown that this method creates more multi-walled nanotubes and some single-walled nanotubes.
Other Carbon Nanotube Synthesis Methods
CNTs are also made by the process called diffusion flame synthesis, heat treatment of a polymer, electrolysis, solar energy use, and low-temperature solid pyrolysis. In flame synthesis, the combustion of a part of the hydrocarbon gas gives the increased temperature needed, with the residual fuel suitable serving as the required hydrocarbon reagent. Thus, the flame establishes an effective source of both hydrocarbon raw material and energy. Combustion synthesis is accessible for high-volume commercial manufacturing.
.png)
Several Carbon Nanotube Synthesis Methods
Carbon Nanotubes Purification Methods
Purification of CNTs refers to the parting of CNTs from their entities, like amorphous carbon, carbon nanoparticles, residual catalyst, and other unwanted species. The conventional chemical techniques for purification have been tested. Yet, they are not effective in the removal of undesirable impurities. There are three methods utilized with diverse levels of success. These include gas-phase, liquid-phase, and intercalation methods. Recently, a new method has been used – plasma purification.
Importantly, a centrifugal parting is important to focus the single-walled nanotubes in low-yield soot prior to the micro filtration operation. This is because nanoparticles easily contaminate membrane filters. The benefit of this process is that unwanted nanoparticles and amorphous carbon are eradicated instantaneously and the CNTs are not chemically changed. But, 2-3 mol nitric acid is valuable for chemically removing impurities.
A classic purification process is as follows: 1 kg CNTs in 20 kgs of 20% HNO3 solution at 80-90 oC for 6hrs, then it is recurrently filtered, DI water washed, and filtered until the filtrate solution becomes pH neutral. The CNTs are dried until the time they form a cake. It is then broken up into fine powder. Persistent sonication lead to the damage of the CNTs structure because of the potent and harsh acids being used.
It is possible to cut CNTs into smaller parts, by prolonged sonication in concentrated acid mixtures. The subsequent CNTs make a colloidal suspension in solvents. These can be collected on substrates or further handles in solution, and can have several different functional groups bounded to the ends and sides of the CNTs.
Gas Phase Carbon Nanotubes Purification
Thomas Ebbesen and his coworkers developed the first successful method for purification of nanotubes. The workers realized that nanoparticles, with their defect rich structures, can be oxidized more freely than the relatively perfect nanotubes following the demonstration that nanotubes could be selectively attached by oxidizing gases. They discovered a vital relative enrichment of nanotubes could be attained this way, but only at the expense of losing the majority of the original sample.
Scientists at the NASA Glenn Research Center created a new gas-phase method to purify gram-scale quantities of single-wall CNTs. The method that involves a modification of the gas-phase purification technique formerly reported by the Smalley and others, utilizes a mixture of high-temperature oxidations and recurrent extractions with nitric and hydrochloric acid. The enhanced procedure considerably decreases the amount of impurities like the residual catalyst, and non-nanotube forms of carbon within the CNTs, boosting their stability dramatically.
Liquid Phase Carbon Nanotubes Purification Methods
The recent liquid-phase purification procedures follow these steps:
- preliminary filtration- to remove huge graphite particles;
- dissolution- to eradicate fullerenes (in organic solvents) and catalyst particles (in concentrated acids)
- centrifugal separation
- microfiltration
- chromatography to either isolate multi-walled nanotubes and unwanted nanoparticles or single-walled nanotubes and the amorphous carbon impurities.
It is essential to keep the CNTs well-separated in the solution, for the CNTs are generally distributed using a surfactant before the last stage of separation.
Intercalation Carbon Nanotubes Purification Methods
Another approach of purification of multi-walled nanotubes was first seen in 1194 by a Japanese research team. The technique used the fact that nanoparticles and other graphitic contaminants have fairly “open” structures and thus, can be more freely intercalated with a diverse range of materials that can close nanotubes.
By introducing with copper chloride, and then decreasing this to metallic copper, the study team was able to differently oxidize the nanoparticles away, utilizing copper as an oxidation catalyst. From the year 1994, this has become a well-known process for purification of nanotubes. The samples of cathodic soot, which have been preserved this way contains almost totally of nanotubes. A drawback of this method is that some amount of nanotubes is unavoidably lost in the oxidation stage, and the final material may be contaminated with residues of intercalates. A parallel purification technique, which involves intercalation with bromine followed by oxidation, has also been demonstrated.
Plasma Purification
Plasma purification is a newer method. Aside from purifying the carbon nanotubes in Argon, the plasma process can be utilized to covalently bond certain functional groups to the nanotubes surface, such as OH, COOH, NH1, N2 & F groups. The plasma method also exfoliates the carbon nanotube material, making it more freely dispersed.
.jpeg)
An Illustration of a Plasma Ball, Similar to Plasma Purification of Nanotubes
Carbon Nanotubes Dispersion
To scatter CNTs, the following process is recommended using the Sonics VCX 750 or equivalent.
It was found that adding sonicating the mixture for 80% of the total time prior to the adding of the dispersant into the solution can improve the dispersion effect by first, dispersing the carbon nanotubes before the surfactant being added to provide stabilization. The reagent, polyvinylpyrrolidone (PVP), is a good dispersion agent. Some individuals like to use the reagent Sodium Dodecyl Benzene Sulfonate (SDBS), Triton 100, or Sodium Dodecyl Sulfonate (SDS). Cheap Tubes has a patent pending distribution method that uses novel nanoscale materials in lieu of conventional surfactants. It does not need to be washed many times to remove extra surfactants and it can be decreased to enhance conductivity in the end product.
The solution consists of CNTs, PVP, and water. The needed sonication time is 30 to 60 minutes with an interruption of 30 seconds every 30 seconds to avoid the breakage of CNT. You must lengthen the time of sonication properly if the power of your ultrasonic equipment is less than that of the SONICS VCX750 unit.
Carbon Nanotubes Functionalization Methods
Pristine nanotubes are inappropriately insoluble in various liquids like polymer resins, water, and most solvents. Hence, they are hard to consistently disperse in a liquid matrix like epoxies and other polymers. This complicates efforts to use the nanotubes’ remaining physical properties in the production of composite materials and also in other practical applications that need the preparation of even mixtures of CNTs with several organic, inorganic, and polymeric materials.
To make nanotubes disperse more easily in liquids, it is important to manually or chemically attach specific molecules or functional groups, to their smooth sidewalls without considerably modifying the nanotubes’ desirable properties. This process is dubbed as functionalization. The manufacture of durable composite materials need robust covalent chemical bonding between the filler particles and the polymer matrix, instead of the weaker van der Waals physical bonds that happen if the CNTs are not appropriately functionalized.
Functionalization methods like oxidation, chopping and wrapping of the CNTs in specific polymers can make more active bonding sites on the surface of the nanotubes. CNTs can be functionalized for biological uses by bonding with biological molecules including lipids, biotins, proteins, etc. Then, they can copy certain biological functions like protein absorption and attach to DNA and drug molecules. This would allow medically and commercially significant applications like gene therapy and drug delivery. Molecules such as carboxylic acid (COOH), poly m-aminobenzoic sulfonic acid (PABS), polyimide, and polyvinyl alcohol (PVA) have been utilized to functionalize CNTs to have amino acid derivatives, compounds and halogens in biochemical and chemical applications like the production of very specific biosensors.
Carbon Nanotubes Functionalization
It is recommended to attach particular chemical functional groups to the carbon nanotube surface to support dispersion in a certain matrix. When the CNTs are not compatible with the matrix, you acquire “islands of CNTs”. This means that your dispersion will have sporadic clear portions in an otherwise homogeneous solution.
The major process of functionalization is by re-fluxing in concentrated acids under high temperatures. This leads to some damage to the sidewalls of the CNTs. However, it has few effects on a multi-walled carbon nanotube because the damage is only on the out walls. The inner walls remain intact.
It can have a drastic effect on the mechanical and conductive properties of single-walled nanotubes. The classical chemical functional groups are hydroxyl – OH, Carboxyl – COOH, & Amine – NH2.
A new method is called microwave plasma based functionalization. During the procedure of plasma based purification, some process gasses are directed into the plasma reactor . When triggered by the energetic plasma, it forms covalent bonds between the surface of the carbon nanotubes and the functional groups. The classical functional groups include OH, COOH, NH2, N2, & F groups.
References
1. “Nanotechnology: Basic Science and Emerging Technologies”, M. Wilson et al, Chapman and Hall (2002) ISBN 1-58488-339-1
2. “Carbon Nanotubes and Related Structures : New Materials for the Twenty-first Century”, P. F. Harris, Cambridge University Press (1999) ISBN 0-521-55446-2
3. “Physical Properties of Carbon Nanotubes”, R. Saito et al, Imperial College Press (1998) ISBN 1-86094-093-5
4. Wondrous World of Carbon Nanotubes (Internet Reference), M. J. M. Daenen et al.
5. Carbon Nanotube Applications (Internet Reference) www.azonano.com/details.asp?ArticleID=980
6. “The Science of Fullerenes and Carbon Nanotubes : Their Properties and Applications”, M. S. Dresselhaus et al, Academic Press (1996) ISBN 0-12221-820-5
7. “Carbon Nanotubes – Preparation and Properties”, T. W. Ebbesen ed., CRC Press (1996) ISBN 0- 84939-602-6
8. “Carbon Nanotubes: Synthesis, Structure, Properties, and Applications”, M. S. Dresselhaus et al eds., Springer-Verlag (2000) ISBN 3-54041-086-4
9. “Carbon Nanotubes”, T. W. Ebbesen, Ann. Rev. Mater. Sci. 24, 235 (1994); Physics Today 381, 678 (1996)
10. [B.Q. Wei, et al, Appl. Phys. Lett. 79 1172 (2001)].
11. [R.H. Baughman, Science 290, 1310 (2000)]
12. [D.Walters, et al., Chem. Phys. Lett. 338, 14 (2001)]
13. [B. Gao, Chem. Phys. Lett. 327, 69 (2000)]
14. [R.Z. Ma, et al., Science in China Series E-Technological Sciences 43 178 (2000)]
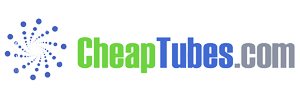
This information has been sourced, reviewed and adapted from materials provided by Cheap Tubes Inc.
For more information on this source, please visit Cheap Tubes Inc.