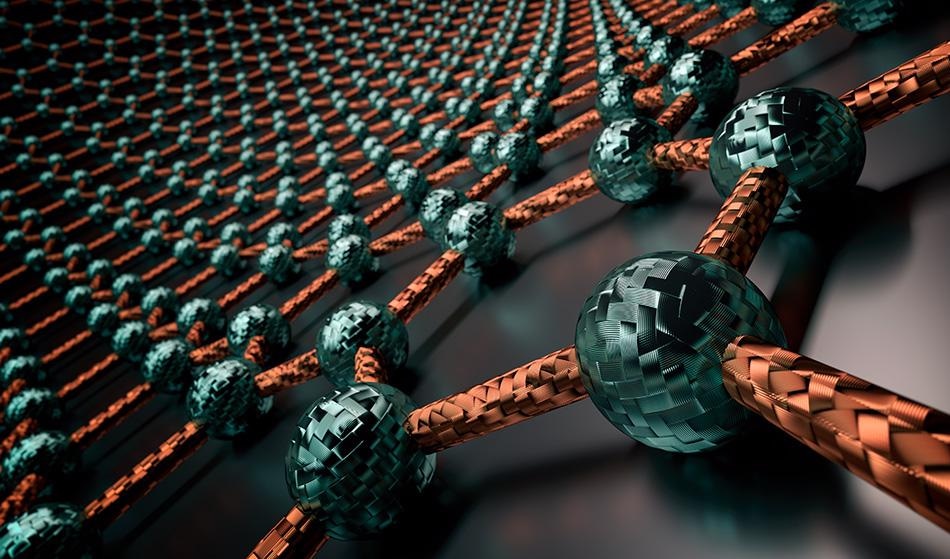
Forance / Shutterstock
The transfer of heat is a vital element in a host of electronic applications today. These include thermoelectrics and optoelectronics such as photodetection, light-harvesting, thermal management, and data communication.
The heat generated while a device operates must be removed to enable the sustained and efficient working of the device over a longer lifetime. For instance, if the electronic components of a microprocessor heat up excessively, the system could degrade in performance and eventually stop working. Complex systems or devices which are built for high-performance standards will build up much more heat, requiring far greater heat fluxes to be dissipated effectively.
How are Heterostructures Helpful in Heat Transfer?
While graphene nanostructures have been promising heat flux candidates due to the high heat conductivity of this material, the cross-plane heat transfer is two orders smaller in magnitude than the in-plane flux, a property called anisotropy.
A new arrival on the scene is van der Waal heterostructures, which are built up of stacked two-dimensional materials of different types forming layers. Thus each layer may be completely different from the next, with interfaces that are crisp and clear at the atomic level.
One such heterostructure is a graphene-copper 3-D microporous heterostructure where copper microparticles are sintered to form a sheet on which graphene is grown. This showed immensely improved heat conduction, with the large surface area enabling the rapid transfer of heat outwards from the structure.
The advantages of enhanced heat transfer using van der Waal heterostructures include the ability to impart better electrical and optoelectronic characteristics and to design innovative device functionalities.
Structure of Graphene-hBN Heterostructures
One van der Waal stack is made up of graphene which is enclosed within hexagonal boron nitride (hBN), which is an insulating two-dimensional material. The mechanism of heat transfer in this material across atomic level planes is via the coupling of electrons (charge carriers) in the graphene with hyperbolic phonon polaritons in the surrounding hBN sheet.
In simple terms, this means that heat flows over picoseconds from the graphene sheet to the encapsulating hBN sheet, at a much faster rate than in-plane transfer, by a couple of orders of magnitudes. This is, therefore, the predominant mechanism of heat transfer.
Mechanism of Heat Transfer
The way in which cross-plane heat transfer occurs is by the coupling of hot electrons in graphene with hyperbolic phonon-polaritons in the hBN. These phonon polaritons are surface modes that allow photon tunneling to occur between metallic surfaces and polar substances.
Photons can tunnel through paths opened by the coupling of evanescent forward- and backward-moving waves from two objects which are nearer to each other than the wavelength of the thermal radiation. In such a case, the number of tunneling photons is much greater than that of propagating photons. This means that heat transfer by radiation is a few orders higher than the black body limit.
The phonon-polaritons travel in the same way as light does in an optical fiber, only their wavelengths are measured in nanometers, which means they fall within the infrared range. Nanostructures have the properties of hyperbolic metamaterials, which means waves can propagate within them with large wave vectors to increase the magnitude of photon tunneling. The hyperbolic wave modes make them capable of carrying heat away very fast.
Resonances are abundant when 2-D materials like graphene are used, which enhance photon tunneling.
With the graphene-hBN heterostructure, for instance, hBN is a natural hyperbolic material and therefore possesses several orders of phonon polariton modes, which will increase the magnitude of photon tunneling. This causes greater heat flux. Thus the use of graphene with other materials to form heterostructures promises to be extremely useful in the transfer of heat in many applications.
Sources
Disclaimer: The views expressed here are those of the author expressed in their private capacity and do not necessarily represent the views of AZoM.com Limited T/A AZoNetwork the owner and operator of this website. This disclaimer forms part of the Terms and conditions of use of this website.