Interview conducted by Olivia FrostAug 21 2023
In this interview, Laura-Isabelle, the Director of Sales at Photon etc., talks to AzoNano about how spectral imaging can help improve the next generation of solar cells.
What is hyperspectral imaging, and what can be measured using this technique?
Hyperspectral imaging is a non-destructive spectroscopy technique that generates comprehensive three-dimensional datasets, with each pixel containing a distinct spectrum. The X-Y axis conveys spatial information in this method, while the Z axis represents spectral information.
This versatile technique allows for a wide range of optical measurements, including (but not limited to) steady-state and time-resolved photoluminescence (PL), electroluminescence (EL), Raman spectroscopy, standard transmittance, reflectance, as well as darkfield microscopy.
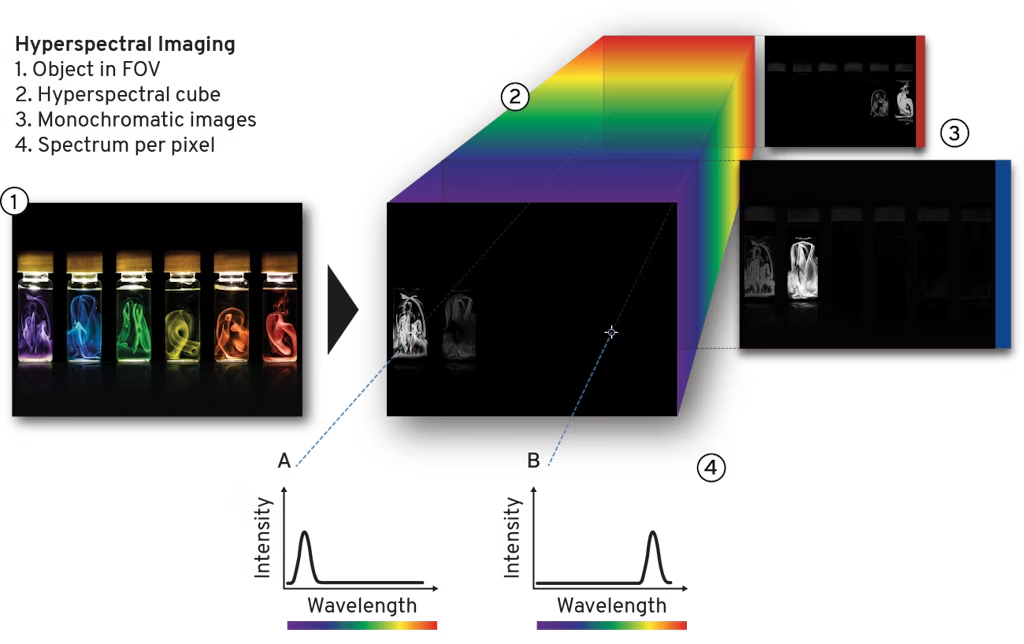
Schematic of a hyperspectral data cube.
Image Credit: Photon etc.
Can you tell us a bit about Photon etc.’s hyperspectral technology? What sets this technology apart from others on the market?
Many hyperspectral systems currently available on the market employ single-point (raster scan) or line-scan (push-broom) imaging techniques. With these approaches, either the samples or the hyperspectral instrument pointing must be moved in order to reconstruct an image.
Photon etc. offers two distinct types of hyperspectral platforms
- The L-EOS: This push-broom hyperspectral camera is specifically designed for in-line inspection purposes.
- The IMA or GRAND-EOS: These global hyperspectral imagers utilize a patented Volume Bragg Grating (VBG) filtering technology that captures monochromatic images sequentially. These platforms were designed with a focus on fundamental research and gaining insights into the optical properties of advanced materials and are particularly suited for the fast acquisition of spectral information from millions of points in the field of view of the camera.
While both imaging capabilities have their advantages and drawbacks, in this interview, we want to focus on the technology behind IMA and the GRAND-EOS, i.e., the global hyperspectral imaging technique.
As mentioned, the filtering technology behind IMA and the GRAND-EOS relies on volume Bragg gratings (VBG). A VBG is a diffraction grating in which there is a periodic modulation of the refractive index through its entire volume. This modulation can be designed to either transmit or reflect the incident beam. In the platforms we developed, VBGs are placed on a rotation stage in the Hypercube (see figure below) in order to select different wavelengths. The Hypercube can be integrated into a standard scientific-grade microscope or combined with standard collection optics. Positioned in front of the sensor, it can accommodate a sCMOS camera, an InGaAs camera, or both, thereby extending the spectral range coverage.
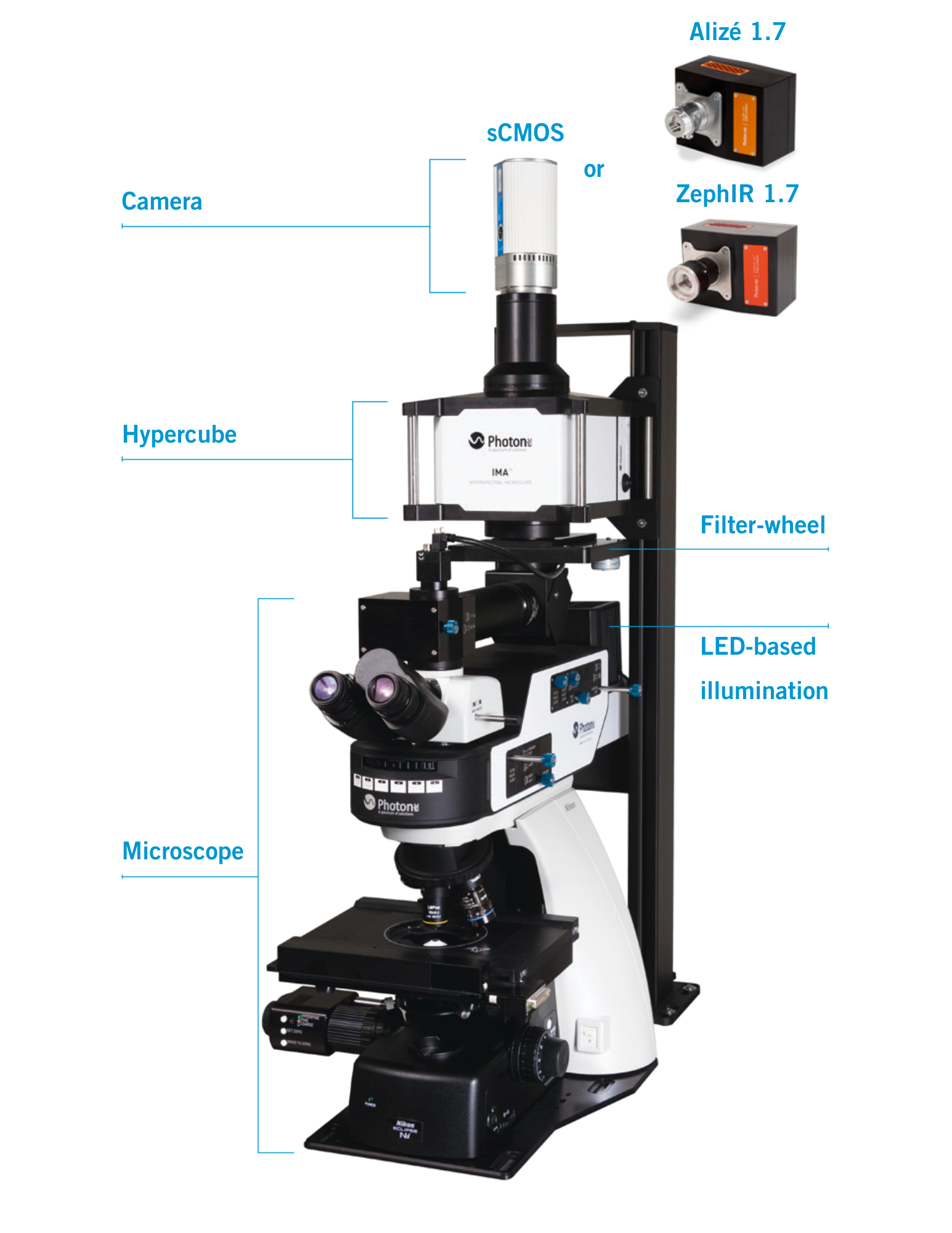
Image Credit: Photon etc.
VBGs allow the filtering of complete images, thereby enabling global imaging. This imaging technique requires uniformly distributing the excitation source (such as LEDs, lasers, white light, etc.) across the entire field of view (FOV) and simultaneously capturing the signal emitted from each individual point within the FOV. This approach eliminates any artifacts due to image reconstruction.
Homogeneous illumination has the added benefit of creating an iso-potential for photogenerated carriers, effectively minimizing their diffusion towards darker, unilluminated regions. This is critical when studying PV materials in complete devices since some operational parameters like charge-depletion width and photoinduced bias depend non-locally on the illumination conditions. Global imaging also enables conducting photoluminescence (PL) experiments within a realistic operating range of 0.1 suns to 500 suns, suitable for PV materials.
Another characteristic that sets our platforms apart is the quantitative nature of the measurements they provide. This means that we can provide photoluminescence (PL) and electroluminescence (EL) data in calibrated units of photon/eV·s·cm2·sr (see example below), rather than relying on arbitrary units. The advantages of absolute calibration will be discussed below.
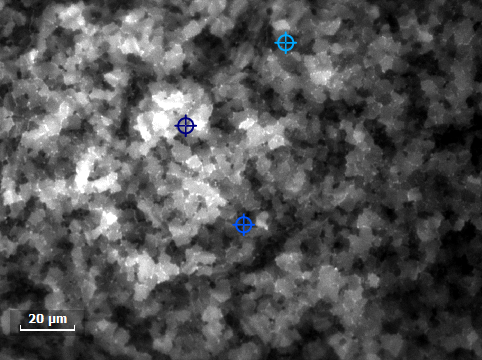
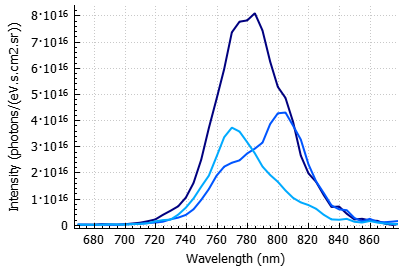
Left: Monochromatic PL image @785 nm of perovskite crystals aged in air excited at 532 nm. Right: Quantitative PL spectra extracted from hyperspectral data.
Image Credit: Photon etc.
What valuable insights do hyperspectral imaging bring to developing novel photovoltaic materials?
Global hyperspectral imaging offers valuable insights into the development of novel photovoltaic candidates by providing detailed spectral and spatial information under realistic conditions of illumination. Before delving into how this can contribute to the development of solar materials, let us first examine the optical techniques employed in this context.
Photoluminescence (PL) and electroluminescence (EL) are well-established and widely recognized non-destructive techniques offering insights into the optical and electronic properties of a given sample, material quality, and device performances. Key pieces of information that PL and EL can provide include material uniformity and composition, carrier generation efficiency and their recombination pathways, bandgap variations, and electronic defects characterization.
Absorbance and reflectance deliver valuable observations on the optical properties and performances of solar materials. Input provided by such techniques includes bandgap determination, optical losses, material uniformity, and light energy conversion efficiency.
Darkfield spectroscopy is a measurement that can offer beneficial information about the scattering and surface properties of photovoltaic materials. Parameters such as surface morphology, scattering mechanisms, light trapping effects, and surface contaminants can be investigated with this method.
Combining hyperspectral imaging with those techniques adds spatial dimensions to the information they provide. This allows the assessment of:
- the spatial distribution of electronic defects, impurities, and variation in material composition;
- the impact of a material’s micro and macrostructure on carrier generation, recombination, and transport;
- the effectiveness of light trapping and surface passivation strategies employed in the device;
All aiding in the optimization of material quality, efficiency, and performance.
It is important to note that most luminescence imaging characterization techniques provide data in arbitrary units, which limits their interpretation because of the lack of information. With this challenge in mind, researchers at IRDEP (now IPVF) have designed a patented method for spectral and photometric calibration.1 This technique has been implemented in Photon etc.’s platform and allows obtaining the absolute number of photons emitted at a specific energy from every point on the sample's surface. By performing this calibration, researchers can further exploit Planck’s law and the reciprocity relations between a solar cell’s External Quantum Efficiency (EQE), its EL emitted at a given voltage, or its PL under a known illumination density.2
Hyperspectral photometric calibrated data contributes to meaningful scientific findings, enabling in-depth interpretation by providing maps of parameters such as:
- Quasi-Fermi Level Splitting (Δμ)1,3
- External Quantum Efficiency (EQE)
- charge transport efficiency3
- saturation current4
- the local voltage of the diode
Beyond these promises, hyperspectral imaging already plays a significant role in guiding the development and improvement of photovoltaic materials by providing essential insights into their optical properties and performance.
For example, in this work by Hamadani et al., they demonstrated that ‘‘hyperspectral luminescence imaging on dilute-alloy InGaAs solar cells can be used to identify and investigate dark spots and the locally high concentration of radiative defects at their center, as well as systemic variations of the luminescence pattern across the device.5
In a Nature publication by Y. Sun et al., worldwide researchers joined forces and demonstrated perovskite light-emitting diodes in the near-infrared spectral range.6 Prof. Sam Stranks’ hyperspectral imaging tool was used to investigate the ‘‘heterogeneities of PL peak wavelength [...], indicating disorder of local energetic structure’’.
How does your technology adjust to the extensive assortment of emerging photovoltaic materials available?
Photon etc.'s hyperspectral imagers are designed with modularity in mind, providing researchers with a versatile tool for their experiments. The intrinsic configuration offers fundamental specifications that facilitate photoluminescence (PL) measurements using high-power LEDs and transmittance measurements in either the visible range (400-1000 nm) or the infrared range (900-1700 nm).
To cater to the specific research projects of different groups, departments, or research centers, an extensive selection of add-ons is available (see figure below).
The instrument's spectral range extension from 400 to 1700 nm enables the characterization of a wide range of candidate materials for next-generation solar cells. The use of sensitive sCMOS and InGaAs cameras allows even the faintest of signals to be observed.
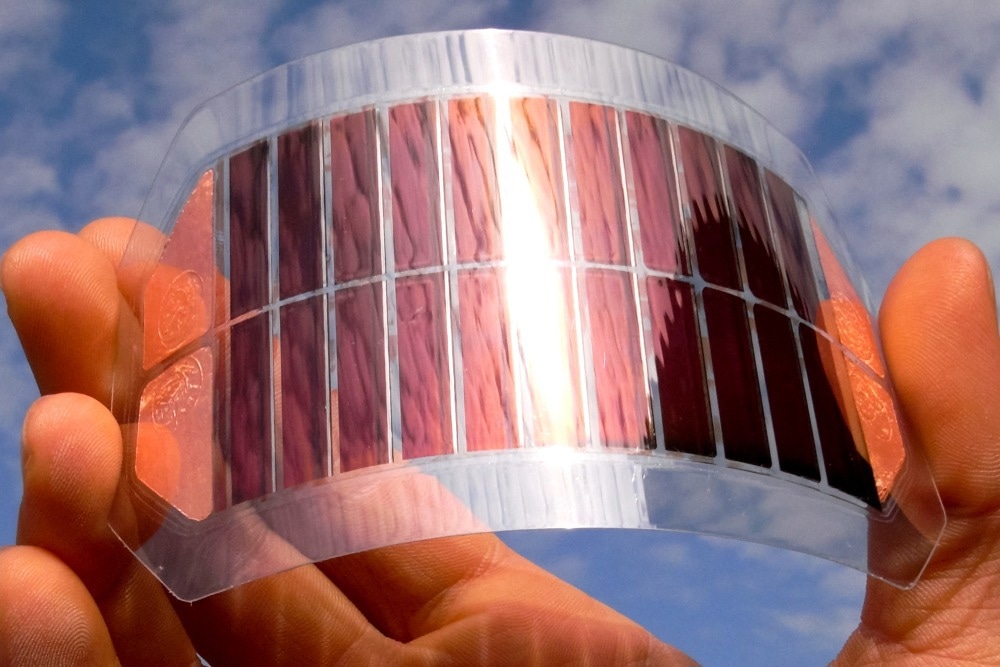
Image Credit: ShutterStock/LuYago
The system supports the integration of multiple excitation wavelengths on a single platform, allowing the investigation of different band-gap materials and tandem cells. For applications requiring higher power density, high-power lasers can be added instead of LEDs. While LEDs are limited to one sun equivalent power at the sample, laser-based illumination can reach dozens or hundreds of suns.
To enhance functionality, a motorized stage can be included, enabling automated measurements and stitching capabilities to accommodate different sizes of samples or devices. Using Photon etc. ’s proprietary software, users can program complex experimental sequences that will be executed automatically by the system without requiring any intervention from lab personnel.
Additional options offered include:
- Absolute calibration module (providing the advantages described in question 3).
- Polarization measurements.
- Epifluorescence module.
- Electroluminescence station, including probes and source meter all integrated within our acquisition software
- Darkfield module.
These various options allow researchers to customize the hyperspectral imager to suit their specific needs, thereby facilitating a wide range of scientific investigations in an efficient and streamlined manner.
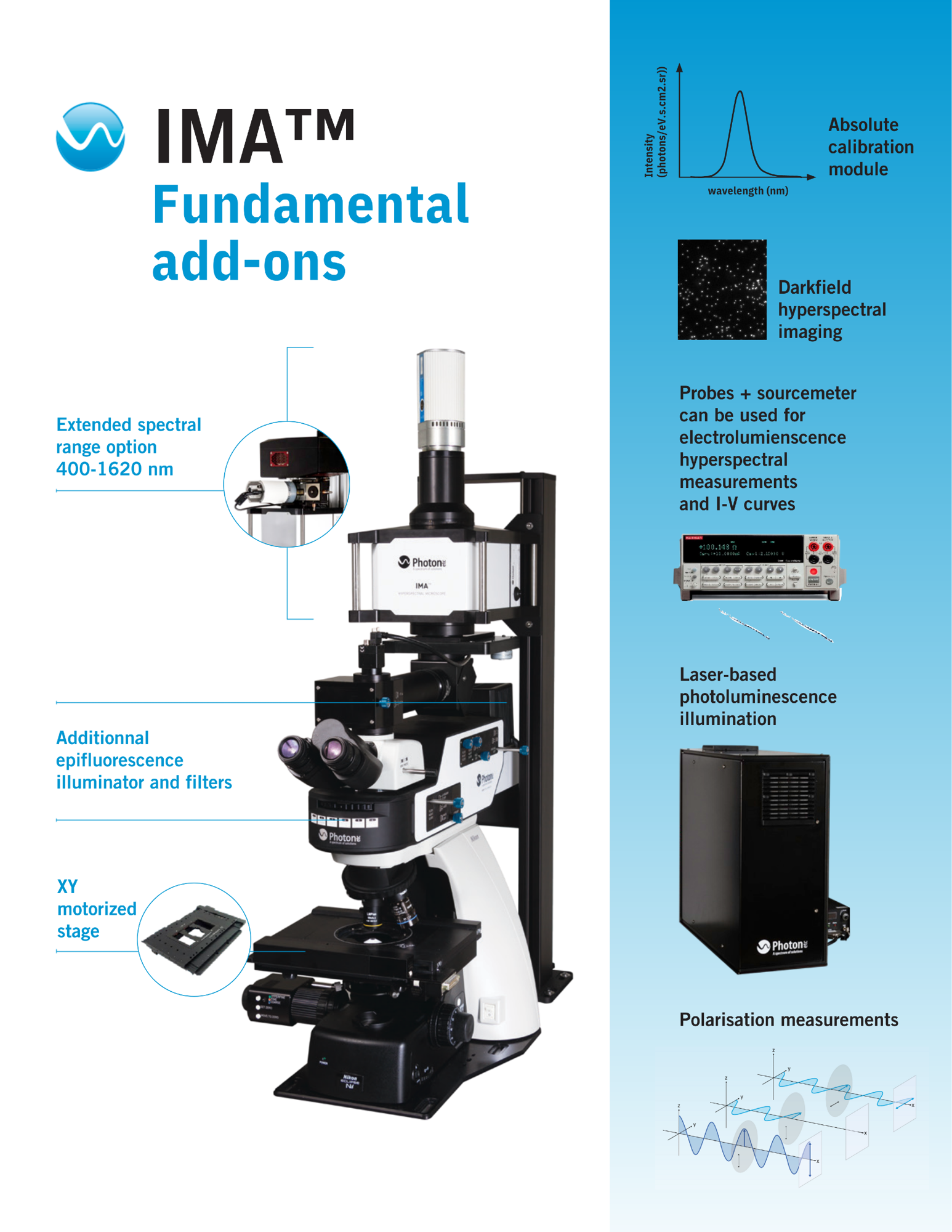
Image Credit: Photon etc.
How can the integration of hyperspectral imaging be effectively incorporated into various stages of the development process within the field of solar cells?
During the initial stages of material development, hyperspectral microscopy enables a deeper understanding of fundamental properties at a microscopic scale. By providing maps of defect distribution, composition homogeneity, photoluminescence quantum yield, and other optoelectronic properties, hyperspectral imaging aids in selecting the optimal fabrication method and enhancing the efficiency of photovoltaic candidates. This comprehensive analysis is precisely what our IMA system delivers, empowering researchers with valuable insights.
As solar materials progress from small-scale devices to standardized modules, the fabrication processes must be scaled up while ensuring reproducibility and reliability. Here, spectral analysis at a larger scale becomes crucial in assessing process uniformity and overall module performance. Moreover, EL and PL are the tools of choice to assess the degradation of modules during environmental stress testing or regular field use. Our GRAND-EOS system is designed precisely for this purpose, facilitating spectral analysis at a larger scale (2x2 cm2, 22x22 cm2) to validate material uniformity, optimize fabrication processes, assess production quality, and track module degradation.
By effectively integrating hyperspectral imaging at every stage of solar cell development, scientists and engineers directly observe the impact of their design strategies at both the microscale and the module level. Doing so provides direct feedback on the effectiveness of these strategies, accelerating the advancement of solar cell technologies. Ultimately, this contributes to the development of efficient, reliable, and commercially viable solar energy solutions.
What are the next development steps for your platform?
Our roadmap is based on a collaboration between Photon etc., and our customers, the researchers developing the next generation of solar materials or the industry commercializing photovoltaic solutions.
A time-resolved photoluminescence (TRPL) hyperspectral imaging platform is currently being investigated by our team. TRPL analysis of solar materials offers crucial information about carrier dynamics, defect characteristics, material quality, charge carrier transport, and interface properties. The synergistic approach of combining TRPL and imaging capabilities will allow for a comprehensive understanding of the material's optical and electrical properties, facilitating the identification of performance-limiting factors, the optimization of device designs, and the development of more efficient solar cells.
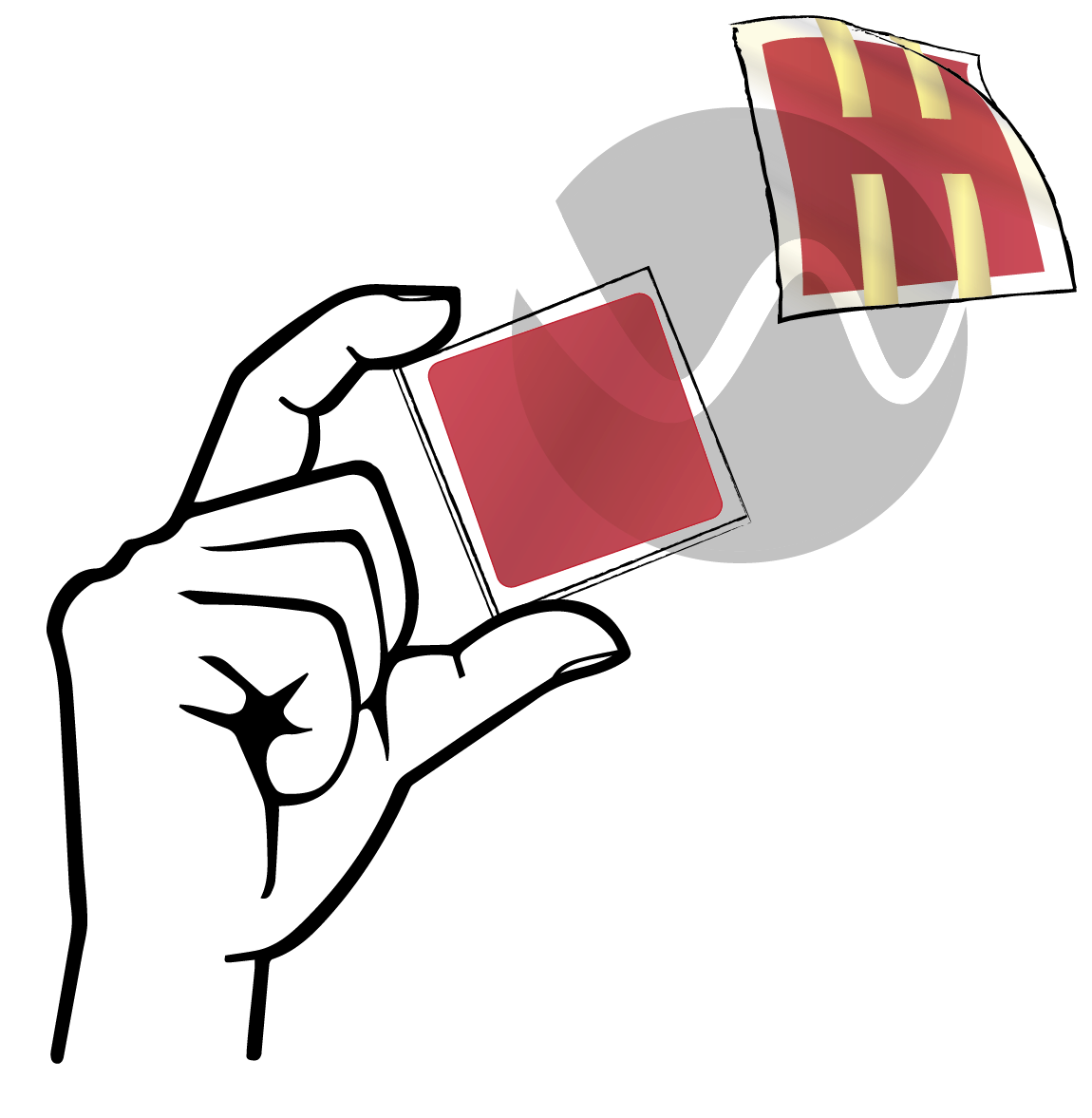
Image Credit: Photon etc.
We’ve also recently added a cryogenic option to our hyperspectral microscopy platform. Cryogenic spectroscopic measurements of solar materials offer a temperature-dependent analysis, the suppression of thermally activated processes, and reduce spectral broadening.
Lastly, we are currently developing a quality control tool to address an essential aspect of solar panel production. As solar panels reach the market, it becomes crucial to incorporate non-destructive, in-line quality control techniques to ensure that the final products meet the desired specifications. Our L-EOS, originally designed for core drill in-line inspection, can be easily adapted to the field of photovoltaics, providing an efficient and reliable solution for quality control. With the L-EOS, real-time monitoring and inspection can be conducted, ensuring the overall performance and compliance of the panels.
Where can our readers go to find out more?
Readers can find additional information on our website dedicated section to solar materials:.
Our products dedicated to material sciences are also available here.
Finally, the list of peer-reviewed scientific papers in the field of photovoltaics can be found here.
About Laura-Isabelle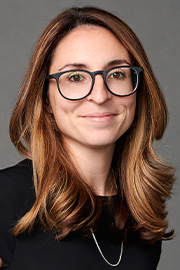
Laura-Isabelle serves as the Director of Sales at Photon, overseeing and guiding the sales team toward achieving their objectives. In addition to her sales leadership, she plays a pivotal role in strategic development and marketing initiatives to drive the company's growth and success.
Laura-Isabelle holds a Bachelor of Science degree in Physics from the Université de Montréal. She further pursued her academic journey at the same institution, obtaining a master's degree in Condensed Matter Physics with a research focus on charge separation in organic photovoltaics.
Her profound expertise in material science has resulted in numerous publications in collaboration with esteemed researchers worldwide. Laura-Isabelle's in-depth knowledge has unlocked new potential avenues for Photon etc., 's cutting-edge hyperspectral imaging systems.
About Photon etc.
Photon etc. is a renowned Canadian-Quebec company, located in the heart of Montreal's innovative district, specializing in the design and manufacturing of hyperspectral and infrared imaging systems. Founded in 2003, our expertise in photonics allows us to provide innovative solutions to the most complex challenges of research and advanced industrial applications.
Scientists and industry alike turn to our expertise for the most advanced imaging and analysis instruments, whether it's to discover new materials for solar energy, see-through living tissue, or obtain the best characterization of an industrial product. This is reflected in the growing number of scientific publications that use our products for their research.
We focus our efforts on three specific sectors: industrial, advanced materials, and life sciences.
We want to hear more about your research. Contact us to start a discussion: [email protected].
References
1. Delamarre A. , Paire M., Guillemoles J.-F. and Lombez L., Quantitative luminescence mapping of Cu(In,Ga)Se2 thin-film solar cells, Progress in Photovoltaics, 2014.
2. Rau, U., Reciprocity relation between photovoltaic quantum efficiency and electroluminescent emission of solar cells, Physical Review B 76(8), 2007.
3. El-Hajje, G., Momblona, C., Gil-Escrig, L., Ávila, J., Guillemot, T., Guillemoles, J.-F., Sessolo, M., Bolink, H. J., & Lombez, L. (2016). Quantification of spatial inhomogeneity in perovskite solar cells by hyperspectral luminescence imaging. In Energy & Environmental Science (Vol. 9, Issue 7, pp. 2286–2294). Royal Society of Chemistry (RSC). https://doi.org/10.1039/c6ee00462h
4. Delamarre, A., Lombez, L., & Guillemoles, J.-F. (2012). Contactless mapping of saturation currents of solar cells by photoluminescence. In Applied Physics Letters (Vol. 100, Issue 13). AIP Publishing. https://doi.org/10.1063/1.3697704
5. Conrad, B., & Hamadani, B. H. (2023). Identifying and investigating spatial features in InGaAs solar cells by hyperspectral luminescence imaging. In AIP Advances (Vol. 13, Issue 3, p. 035318). AIP Publishing. https://doi.org/10.1063/5.0131691
6. Sun, Y., Ge, L., Dai, L., Cho, C., Ferrer Orri, J., Ji, K., Zelewski, S. J., Liu, Y., Mirabelli, A. J., Zhang, Y., Huang, J.-Y., Wang, Y., Gong, K., Lai, M. C., Zhang, L., Yang, D., Lin, J., Tennyson, E. M., Ducati, C., … Greenham, N. C. (2023, March 15). Bright and stable perovskite light-emitting diodes in the near-infrared range. Nature News. https://www.nature.com/articles/s41586-023-05792-4
Disclaimer: The views expressed here are those of the interviewee and do not necessarily represent the views of AZoM.com Limited (T/A) AZoNetwork, the owner and operator of this website. This disclaimer forms part of the Terms and Conditions of use of this website.