Scientists at the National Graphene Institute have made a significant breakthrough that has the potential to transform energy capture and information processing. Their research, detailed in a paper in Nature, demonstrates the ability of electric field effects to selectively enhance coupled electrochemical reactions within graphene.
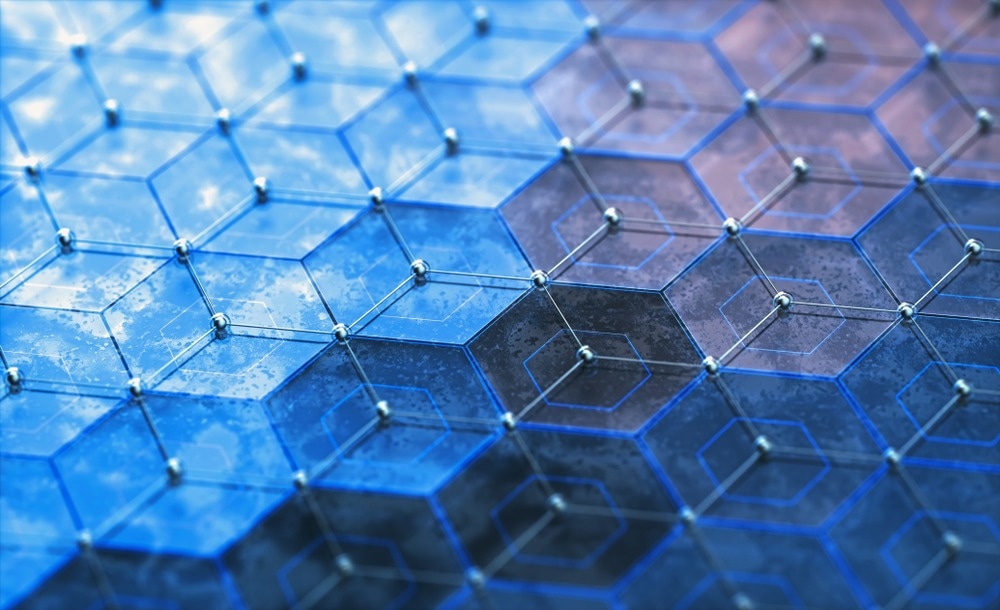
Image Credit: ktsdesign/Shutterstock.com
Batteries, fuel cells, and electrolyzers are renewable energy technologies that depend on electrochemical processes. However, sluggish reactions and undesirable side effects frequently compromise their effectiveness. Traditional tactics have focused on new materials, yet there are still many obstacles to overcome.
Under the direction of Dr. Marcelo Lozada-Hidalgo, the Manchester team has adopted a unique strategy. They have managed to break the irreversible bond between charge and electric field in graphene electrodes, providing previously unheard-of control over the material's electrochemical reactions. This discovery casts doubt on earlier theories and creates new opportunities for energy technologies.
We have managed to open up a previously inaccessible parameter space. A way to visualize this is to imagine a field in the countryside with hills and valleys. Classically, for a given system and a given catalyst, an electrochemical process would run through a set path through this field. If the path goes through a high hill or a deep valley – bad luck. Our work shows that, at least for the processes we investigated here, we have access to the whole field. If there is a hill or valley we do not want to go to, we can avoid it.
Dr. Marcelo Lozada-Hidalgo, National Graphene Institute
The study focuses on processes related to protons that are essential for electrical devices and hydrogen catalysts. The group specifically looked at two proton reactions in graphene:
Proton Transmission: The development of novel hydrogen catalysts and fuel cell membranes depends on this mechanism.
Proton Adsorption (Hydrogenation): This process turns graphene's conductivity on and off, making it crucial for electronic devices like transistors.
Both processes were historically connected in graphene devices; controlling one without affecting the other was difficult. After successfully separating these processes, the researchers discovered that hydrogenation may be driven independently by electric field effects, which might also dramatically speed up proton transport. This unexpected selective acceleration introduces a novel approach to driving electrochemical processes.
We demonstrate that electric field effects can disentangle and accelerate electrochemical processes in 2D crystals. This could be combined with state-of-the-art catalysts to efficiently drive complex processes like CO2 reduction, which remain enormous societal challenges.
Dr Jincheng Tong, Study First Author, National Graphene Institute
Dr Yangming Fu, Co-First Author, pointed to potential applications in computing: “Control of these process gives our graphene devices dual functionality as both memory and logic gate. This paves the way for new computing networks that operate with protons. This could enable compact, low-energy analog computing devices.”
Journal Reference:
Tong, J., et al. (2024) Control of proton transport and hydrogenation in double-gated graphene. Nature. doi.org/10.1038/s41586-024-07435-8