By Nidhi DhullReviewed by Lexie CornerUpdated on Aug 20 2024
Gene manipulation involves multiple steps, beginning with the introduction of genes of interest into cells and tissues. Various methods are used to deliver deoxyribonucleic acid (DNA) sequences across cell walls, plasma membranes, and nuclear membranes in mammalian, animal, and plant cells.1 However, even advanced gene editing tools like CRISPR (clustered regularly interspaced short palindromic repeat) systems still lack safe and efficient delivery methods.2
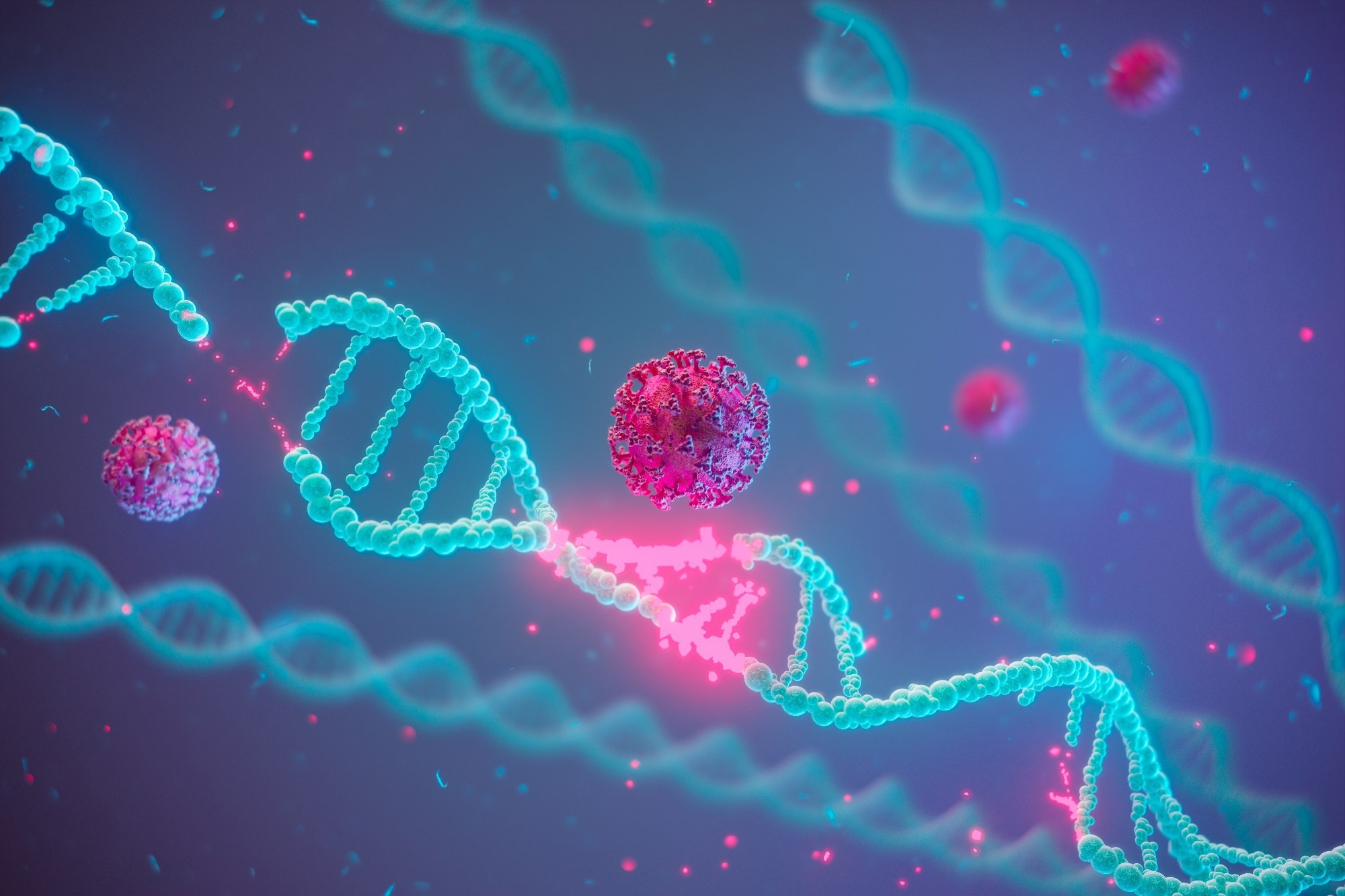
Image Credit: Dabarti CGI/Shutterstock.com
Nanotechnology has emerged as a promising alternative for genetic transformation. Nanoparticles of different shapes and sizes can deliver DNA into living cells, enabling stable integration and gene expression.1 Integrating nanotechnology with biomedicine through functional nanocomposites helps overcome the limitations of CRISPR delivery.2
This article explores the potential implications of nanotechnology for the future of gene manipulation.
Nanotechnology in Genetic Engineering
Gene editing techniques allow precise modifications of plant and animal genes, revolutionizing biomedical sciences. These techniques primarily involve introducing transgenes or CRISPR into plants or animals using specific gene delivery systems.
However, traditional delivery systems are time-consuming, involve complicated protocols, and can damage tissues. In contrast, nanotechnology-based gene delivery offers high efficiency for temporal (transient) and permanent (stable) gene modifications across various species.3
Carbon-based nanoparticles, such as fullerenes and carbon nanotubes, exhibit excellent physical and chemical properties, including high aspect ratio, tensile strength, surface area-to-volume ratio, biocompatibility, and biostability, making them efficient gene carriers. For example, single-walled carbon nanotubes complexed with chitosan can transfer DNA into chloroplasts of mature plants.1
Mesoporous silica nanoparticles (MSNPs) with honeycomb structures are widely used in gene editing for plant and animal cells. They prevent molecule leaching by entrapping them inside pores and encapsulating them. Due to their safety, biodegradability, and biocompatibility, MSNPs effectively deliver genetic materials of various sizes, shapes, and functionalities, including proteins, DNA, and ribonucleic acid.1
Metallic nanoparticles (MNPs), composed of elements like gold, silver, iron oxide, copper oxide, and zinc oxide, readily attract negatively charged DNA molecules due to their positive surface charge. They can deliver DNA into vegetative and reproductive tissues of plants to enhance their functions. For instance, MNP-transfected pollen grains in transgenic cotton plants exhibit resistance against insects.1
Quantum dots, another type of metal-based nanoparticle, have successful applications in gene engineering. For example, ZnS-based plasmid DNA introduced into young tobacco plants effectively integrates the gene of interest into the tobacco genome with stable expression.
In addition, nanoparticles based on organic polymers, lipids, peptides, and vesicles also function as bioactive carriers in gene delivery and editing.1 Polymeric nanoparticles allow the combined application of gene therapy and anticancer drugs or nano agents for photothermal therapy, enhancing targeted delivery and therapeutic potency.2
Nanoparticle-assisted delivery of CRISPR/Cas systems for in vivo genome has been proposed for the liver, where nanoparticles accumulate after systemic administration. Additionally, lipid nanoparticles have shown high success rates in selectively delivering CRISPR payloads to the liver, spleen, lungs, and tumor tissues.
Alternatively, nanotube wire arrays can distribute plasmid DNA to modify living neuronal cells in the brain and pacemaker cells in mammalian circadian rhythms.2
Advantages of Nanotechnology in Gene Manipulation
Conventional gene editing approaches face challenges such as limited host range, low transformation efficiency, tissue damage, and random integration of DNA into the host genome. Additionally, traditional chemical and physical biotransformation methods often involve excessive chemicals and energy.1 In contrast, nanocomposites offer promising advantages for CRISPR/Cas delivery due to their excellent biocompatibility, lower toxicity, and enhanced delivery efficacy.2
The successful expression of exogenous genes requires stable integration to develop transgenic plants. Nanoparticles help maintain the stability of the genes of interest due to their small size and surface effects.1 The tunable physicochemical properties of nanocomposites also allow easy conjugation with various functional materials.2
Nanoparticles can be charged, and their surfaces modified to bind with diverse biomolecules. Engineered nanoparticles enable cargo delivery to subcellular parts, such as mitochondrial or chloroplast DNA, without the species- and tissue-specific limitations of conventional biomolecule delivery methods. For example, nanoparticles can traverse plant cell walls due to their small size.3 Additionally, nanocomposites allow organ-specific delivery of CRISPR/Cas systems.2
Nanotechnology in Gene Manipulation: Challenges and Limitations
Despite the revolutionary potential of nanotechnology in gene editing, its large-scale adoption faces several challenges. Primarily, nano-phytotoxicity can negatively impact plants’ physical and reproductive growth.
Nanomaterials released into the environment can damage plants and ecosystems, and blockages in a plant’s vascular system due to nanomaterials can structurally damage DNA and induce oxidative stress.3
The delivery of CRISPR/Cas tools through nanocomposites has not yet been tested on human tissue-derived organoids, which provide better preclinical modeling by mimicking patients' genetic setups.2 Additionally, nanoparticle-based gene modification has been studied only in a few plants, such as cotton, tobacco, tomato, wheat, lupin, pumpkin, maize, and potato, with limited studies on monocotyledons.1
Different nanomaterials behave differently in specific plant cells, requiring thorough dose optimization and spectral tuning for various species. The biomolecule-binding affinity of nanoparticles varies with their structure, charge, chemical composition, and surface area. Thus, it is crucial to ensure that nanoparticles do not interfere with cellular processes to avoid disrupting cell structural stability and metabolic pathways.3
Future Directions in Nanotechnology for Gene Manipulation
Nanotechnology’s transformative potential in gene manipulation is being explored for novel applications.
For instance, a recent article in Heliyon explored nanotechnology-based genetic engineering for sustainable seaweed cultivation. Macroalgae is a great source of food, fuel, and bio-products, and its farming is gaining popularity as demand for resources grows. However, commercial-scale and sustainable seaweed farming is challenging. Nanoparticles could genetically enhance macroalgae species, helping to realize their full potential.4
Another recent study in the Journal of Drug Delivery Science and Technology highlighted the significance of nanorobots for DNA-based cancer treatment, overcoming chemotherapy's harsh side effects and untargeted drug delivery.
Nanorobots, controlled devices made up of nanometric components, can interact with and diffuse through cellular membranes. This direct access to the cellular level improves cancer treatment efficiency through novel methods like gene therapy, including gene correction, silencing, activation, and editing.5
Overall, nanostructures and nanocomposites hold promise for accelerating the clinical application of gene modification methods, such as CRISPR delivery systems, potentially revolutionizing healthcare.2 They also offer significant advantages in designing smart crop systems, making agriculture more effective, robust, and sustainable in the face of the impending climate crisis.
The existing challenges in nanotechnology-based gene engineering can be addressed by integrating different gene delivery methods, like magnetofection and CRISPR, and fabricating novel hybrid nanomaterials.3
More from AZoNano: Advanced Optical Emission Spectroscopy in Plasma Systems
References and Further Reading
1. Begum, R., Jayawardana, NU. (2021). A review of nanotechnology as a novel method of gene transfer in plants. Journal of Agricultural Sciences. DOI: 10.4038/jas.v16i2.9336, https://jas.sljol.info/articles/10.4038/jas.v16i2.9336
2. Ghani, MW., Iqbal, A., Ghani, H., Bibi, S., Wang, Z., Pei, R. (2023). Recent advances in nanocomposite-based delivery systems for targeted CRISPR/Cas delivery and therapeutic genetic manipulation. Journal of Materials Chemistry B. DOI: 10.1039/d2tb02610d, https://pubs.rsc.org/en/content/articlehtml/2023/tb/d2tb02610d
3. Ahmar, S., et al. (2021). Advantage of Nanotechnology-Based Genome Editing System and Its Application in Crop Improvement. Frontiers in Plant Science. DOI: 10.3389/fpls.2021.663849, https://www.frontiersin.org/journals/plant-science/articles/10.3389/fpls.2021.663849/full
4. Khan, N., Sudhakar, K., Mamat, R. (2023). Seaweed farming: A perspectives of genetic engineering and nano-technology application. Heliyon. DOI: 10.1016/j.heliyon.2023.e15168, https://www.cell.com/heliyon/fulltext/S2405-8440(23)02375-7
5. Chattha, GM., et al. (2023). Nanorobots: An innovative approach for DNA-based cancer treatment. Journal of Drug Delivery Science and Technology. DOI: 10.1016/j.jddst.2023.104173, https://www.sciencedirect.com/science/article/abs/pii/S1773224723000254
Disclaimer: The views expressed here are those of the author expressed in their private capacity and do not necessarily represent the views of AZoM.com Limited T/A AZoNetwork the owner and operator of this website. This disclaimer forms part of the Terms and conditions of use of this website.